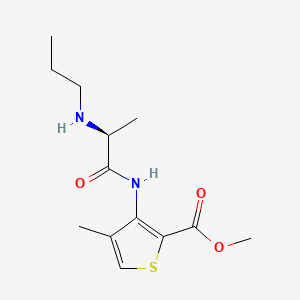
Articaine, (S)-
- Click on QUICK INQUIRY to receive a quote from our team of experts.
- With the quality product at a COMPETITIVE price, you can focus more on your research.
Overview
Description
Articaine (S)-, a fourth-generation amide local anesthetic, is distinguished by its unique thiophene ring and ester side chain (C₁₃H₂₀N₂O₃S; molecular mass: 284.374 g/mol) . Introduced in 1976, it has become the most widely used dental anesthetic in Germany (72% adoption in 1989), Italy, and the Netherlands due to its rapid onset, high efficacy, and favorable safety profile . Articaine’s dual metabolism—hydrolysis by plasma esterases (80–90%) and hepatic enzymes (10–20%)—results in a short elimination half-life (27 minutes), reducing systemic toxicity risks .
Preparation Methods
Articaine, (S)-, can be synthesized through a series of chemical reactions. One common method involves the amidation of 4-methyl-3-aminothiophene-2-methyl formate with 2-chloro propionyl chloride, followed by ammoniation with propylamine, and finally, salification with concentrated hydrochloric acid to obtain articaine hydrochloride . This method is suitable for industrial production due to its simplicity, high yield, and the availability of raw materials .
Chemical Reactions Analysis
Articaine, (S)-, undergoes various chemical reactions, including:
Oxidation: Articaine can be oxidized to form articainic acid, its inactive metabolite.
Reduction: Reduction reactions are less common for articaine due to its stable structure.
Substitution: Articaine can undergo substitution reactions, particularly at the thiophene ring, which can be modified to alter its pharmacological properties. Common reagents used in these reactions include oxidizing agents like hydrogen peroxide and reducing agents like sodium borohydride.
Scientific Research Applications
Pharmacokinetics
Articaine is notable for its rapid metabolism; approximately 90% is hydrolyzed in the bloodstream to form the inactive metabolite articainic acid. This results in a shorter elimination half-life (20-30 minutes) compared to lidocaine (90-120 minutes), contributing to lower systemic toxicity and a wider therapeutic range .
Property | Articaine | Lidocaine |
---|---|---|
Half-life | 20-30 minutes | 90-120 minutes |
Metabolism | Rapid hydrolysis | Hepatic metabolism |
Primary Metabolite | Articainic acid | Monoethylglycinexylidide |
Protein Binding | High | Moderate |
Clinical Applications
Articaine's unique properties make it particularly advantageous in various clinical settings:
- Dental Procedures : Articaine is preferred for dental anesthesia due to its rapid onset and effective pain control. Studies indicate higher success rates in achieving profound anesthesia compared to lidocaine .
- Pediatric Dentistry : Its safety profile makes it suitable for use in children, where it has been shown to provide effective anesthesia with fewer injections required .
- Geriatric Patients : Due to its favorable metabolic pathway, Articaine can be advantageous for older patients who may have liver dysfunction .
Comparative Efficacy
Numerous studies have compared the efficacy of Articaine with other local anesthetics:
- Mandibular Anesthesia : A systematic review found that 4% Articaine had a success rate 1.37 times greater than 2% lidocaine for mandibular teeth .
- Maxillary Buccal Infiltration : For maxillary procedures, Articaine showed a success rate slightly higher than lidocaine but not statistically significant .
- Endoscopic Procedures : In endoscopic endonasal surgeries, Articaine demonstrated significantly lower pain scores and higher anesthesia efficacy compared to lidocaine .
Case Studies
Several case studies have highlighted the effectiveness of Articaine in clinical settings:
- Efficacy in Dental Anesthesia : A study involving 180 patients demonstrated that Articaine provided a significantly higher effective proportion (89%) compared to lidocaine (76.6%) during dental procedures .
- Impact on Metabolic Activity : A comparative metabolomics study assessed the effects of Articaine on neuronal cells, finding alterations in metabolic patterns indicative of potential neuroprotective effects alongside its anesthetic properties .
Mechanism of Action
Articaine, (S)-, exerts its effects by blocking the generation and conduction of nerve impulses. It increases the threshold for electrical excitation in the nerve, slows the propagation of the nerve impulse, and reduces the rate of rise of the action potential . This action is primarily due to its ability to bind to and inhibit voltage-gated sodium channels in the neuronal membrane .
Comparison with Similar Compounds
Chemical and Pharmacokinetic Properties
Parameter | Articaine (S)- | Lidocaine | Bupivacaine | Prilocaine |
---|---|---|---|---|
Chemical Structure | Thiophene ring | Benzene ring | Benzene ring | Benzene ring |
pKa | 7.8 | 7.7 | 8.1 | 7.9 |
Protein Binding (%) | 95 | 65 | 95 | 55 |
Half-Life (min) | 27 | 90 | 210 | 90 |
Metabolism | Plasma esterases | Liver (CYP450) | Liver (CYP450) | Liver (CYP450) |
Articaine’s thiophene ring enhances lipid solubility, improving tissue diffusion compared to lidocaine’s benzene ring . Its lower pKa (7.8 vs. 8.1 for bupivacaine) ensures faster nerve membrane penetration in inflamed tissues .
Clinical Efficacy
- Onset and Duration: Articaine (4% with 1:200,000 epinephrine) exhibits an onset of 1.5–1.8 minutes for maxillary infiltration, comparable to lidocaine . However, in third molar surgeries, articaine provided a longer duration (4.28 hours vs. 3.51 hours for lidocaine) . A 2024 study in endoscopic endonasal dacryocystorhinostomy (EN-DCR) demonstrated superior pain control with 4% articaine vs. 2% lidocaine, attributed to higher concentration and vasoconstrictor synergy .
Pediatric Use :
Articaine infiltrations in primary molars reduce the need for inferior alveolar nerve blocks, minimizing post-treatment soft tissue injuries in children over 4 years .
Market Perception vs. Evidence
- Clinical Adoption: Despite meta-analyses confirming articaine’s superiority over lidocaine for routine dental procedures, 40% of practitioners avoid it for inferior alveolar blocks due to outdated perceptions .
Biological Activity
Articaine (S) is a local anesthetic widely used in dental procedures and other medical applications. It is classified as an amide local anesthetic with unique pharmacological properties that enhance its efficacy and safety profile. This article explores the biological activity of Articaine (S), focusing on its pharmacodynamics, pharmacokinetics, and clinical applications, supported by research findings and case studies.
Articaine is characterized by its thiophene ring structure, which enhances lipid solubility compared to traditional amide local anesthetics such as lidocaine. This structural feature allows for better diffusion through nerve membranes, leading to increased potency and efficacy in nerve block procedures .
The mechanism of action involves reversible binding to the α-subunit of voltage-gated sodium channels in nerve cells. This binding inhibits sodium influx, preventing the generation and propagation of action potentials, thereby producing anesthesia . Articaine exhibits state-dependent binding, with higher affinity for open and inactivated states of the sodium channel .
Pharmacokinetics
Articaine's pharmacokinetics are notable for its rapid metabolism. Approximately 90% of the drug is hydrolyzed in the bloodstream to form the inactive metabolite articainic acid, which is then excreted via the kidneys. This rapid metabolism results in a shorter elimination half-life of about 20-30 minutes, compared to 90-120 minutes for lidocaine . The presence of an ester group allows for this quick hydrolysis, contributing to lower systemic toxicity and a wider therapeutic range .
Table 1: Pharmacokinetic Properties of Articaine vs. Lidocaine
Property | Articaine | Lidocaine |
---|---|---|
Half-life | 20-30 minutes | 90-120 minutes |
Metabolism | Rapid hydrolysis | Hepatic metabolism |
Primary Metabolite | Articainic acid | Monoethylglycinexylidide |
Protein Binding | High | Moderate |
Clinical Applications
Articaine's unique properties make it particularly useful in various clinical settings:
- Dental Procedures : Articaine is preferred for dental anesthesia due to its rapid onset and effective pain control. Studies indicate higher success rates in achieving profound anesthesia compared to lidocaine .
- Pediatric Dentistry : Its safety profile makes it suitable for use in children, where it has been shown to provide effective anesthesia with fewer injections required .
- Geriatric Patients : Due to its favorable metabolic pathway, articaine can be advantageous for older patients who may have liver dysfunction .
Case Studies
- Efficacy in Dental Anesthesia : A study involving 180 patients demonstrated that articaine provided a significantly higher effective proportion (89%) compared to lidocaine (76.6%) during dental procedures. The visual analog scale (VAS) scores indicated less pain perception in the articaine group (4.4 cm) versus the lidocaine group (5.0 cm), highlighting its superior efficacy .
- Impact on Metabolic Activity : A comparative metabolomics study assessed the effects of articaine on SH-SY5Y neuronal cells. The study found that exposure to articaine significantly altered metabolic patterns, including decreased glucose consumption and lactate secretion, indicating potential neuroprotective effects alongside its anesthetic properties .
Safety Profile
While articaine is generally considered safe, it is essential to monitor patients for potential adverse effects such as transient neurological symptoms or allergic reactions. Its lower toxicity compared to other amide anesthetics makes it a favorable choice for many clinical applications; however, caution is advised when used in patients with known sensitivities or genetic variations affecting drug metabolism .
Q & A
Basic Research Questions
Q. What are the best practices for synthesizing and characterizing (S)-Articaine to ensure reproducibility in pharmacological studies?
- Methodological Answer :
- Synthesis : Use enantioselective synthesis techniques, such as asymmetric catalysis or chiral auxiliary approaches, to isolate the (S)-enantiomer. Document reaction conditions (temperature, solvent, catalysts) meticulously .
- Characterization : Employ nuclear magnetic resonance (NMR) spectroscopy for structural confirmation, high-performance liquid chromatography (HPLC) with chiral columns for enantiomeric purity assessment (>98%), and mass spectrometry (MS) for molecular weight validation. Provide raw spectral data in supplementary materials .
- Reproducibility : Include step-by-step protocols for synthesis and purification in the main manuscript or supplementary files, adhering to journal guidelines for experimental transparency .
Q. How should researchers design in vitro models to evaluate the local anesthetic efficacy of (S)-Articaine compared to its racemic form?
- Methodological Answer :
- Cell-Based Assays : Use voltage-clamp techniques on sodium channels (e.g., Nav1.7) in dorsal root ganglion neurons to measure inhibition potency (IC₅₀). Normalize results against racemic Articaine and lidocaine controls .
- Tissue Models : Conduct ex vivo nerve block experiments on isolated sciatic nerves (e.g., rat models) to compare onset time and duration of action. Ensure blinding and randomization to reduce bias .
- Statistical Analysis : Apply ANOVA with post-hoc Tukey tests to assess significance (p < 0.05) and report effect sizes .
Advanced Research Questions
Q. What strategies can resolve contradictions in reported pharmacokinetic data for (S)-Articaine across different preclinical studies?
- Methodological Answer :
- Meta-Analysis : Systematically review existing studies using PRISMA guidelines. Stratify data by species, administration route (e.g., subcutaneous vs. intravenous), and analytical methods (e.g., LC-MS/MS vs. ELISA) to identify confounding variables .
- In Silico Modeling : Develop physiologically based pharmacokinetic (PBPK) models to simulate interspecies differences in metabolism and clearance. Validate predictions with targeted in vivo experiments .
- Data Transparency : Share raw datasets and computational code via open-access repositories to enable independent verification .
Q. How can researchers ensure enantiomeric purity of (S)-Articaine in long-term stability studies under varying storage conditions?
- Methodological Answer :
- Stability Protocols : Store samples at controlled temperatures (4°C, 25°C, 40°C) and humidity levels (e.g., 60% RH). Test enantiomeric purity at intervals (0, 3, 6, 12 months) using chiral HPLC .
- Degradation Analysis : Identify degradation products via LC-MS and compare fragmentation patterns to known impurities. Quantify using calibration curves with ≥5-point linearity (R² > 0.99) .
- Statistical Reporting : Use accelerated stability models (e.g., Arrhenius equation) to predict shelf life and report 95% confidence intervals .
Q. What computational approaches are optimal for predicting the binding affinity of (S)-Articaine to neuronal sodium channels?
- Methodological Answer :
- Molecular Dynamics (MD) : Simulate ligand-receptor interactions using cryo-EM structures of Nav1.7 (e.g., PDB ID 6J8E). Calculate binding free energy with MM-GBSA or umbrella sampling .
- Docking Studies : Perform blind docking with AutoDock Vina to identify putative binding sites. Validate with mutagenesis data (e.g., residues F1749 and E1553 in Nav1.7) .
- Machine Learning : Train QSAR models on published IC₅₀ values of sodium channel blockers to predict (S)-Articaine’s efficacy. Use k-fold cross-validation to avoid overfitting .
Q. Guidance for Addressing Methodological Challenges
- Contradictory Data : Apply sensitivity analysis to assess the impact of experimental variables (e.g., pH, solvent polarity) on outcomes .
- Ethical Reproducibility : Pre-register study designs on platforms like Open Science Framework and adhere to ARRIVE guidelines for animal research .
- Literature Gaps : Use tools like SciFinder or Reaxys to map citation networks and identify understudied areas (e.g., metabolite neurotoxicity) .
Properties
CAS No. |
1443683-48-4 |
---|---|
Molecular Formula |
C13H20N2O3S |
Molecular Weight |
284.38 g/mol |
IUPAC Name |
methyl 4-methyl-3-[[(2S)-2-(propylamino)propanoyl]amino]thiophene-2-carboxylate |
InChI |
InChI=1S/C13H20N2O3S/c1-5-6-14-9(3)12(16)15-10-8(2)7-19-11(10)13(17)18-4/h7,9,14H,5-6H2,1-4H3,(H,15,16)/t9-/m0/s1 |
InChI Key |
QTGIAADRBBLJGA-VIFPVBQESA-N |
Isomeric SMILES |
CCCN[C@@H](C)C(=O)NC1=C(SC=C1C)C(=O)OC |
Canonical SMILES |
CCCNC(C)C(=O)NC1=C(SC=C1C)C(=O)OC |
Origin of Product |
United States |
Disclaimer and Information on In-Vitro Research Products
Please be aware that all articles and product information presented on BenchChem are intended solely for informational purposes. The products available for purchase on BenchChem are specifically designed for in-vitro studies, which are conducted outside of living organisms. In-vitro studies, derived from the Latin term "in glass," involve experiments performed in controlled laboratory settings using cells or tissues. It is important to note that these products are not categorized as medicines or drugs, and they have not received approval from the FDA for the prevention, treatment, or cure of any medical condition, ailment, or disease. We must emphasize that any form of bodily introduction of these products into humans or animals is strictly prohibited by law. It is essential to adhere to these guidelines to ensure compliance with legal and ethical standards in research and experimentation.