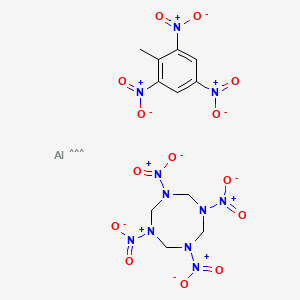
Octonal
- Click on QUICK INQUIRY to receive a quote from our team of experts.
- With the quality product at a COMPETITIVE price, you can focus more on your research.
Overview
Description
Octanol, also known as octan-1-ol, is an organic compound with the molecular formula C₈H₁₈O. It is a fatty alcohol and is one of the many isomers known generically as octanols. Octanol is a colorless liquid with a characteristic aromatic odor and is used in the synthesis of esters for perfumes and flavorings .
Preparation Methods
Synthetic Routes and Reaction Conditions
Octanol is primarily produced industrially by the oligomerization of ethylene using triethylaluminium followed by oxidation of the alkylaluminium products. . The reaction can be summarized as follows: [ \text{Al(C}_2\text{H}_5\text{)}_3 + 9 \text{C}_2\text{H}_4 \rightarrow \text{Al(C}8\text{H}{17}\text{)}_3 ] [ \text{Al(C}8\text{H}{17}\text{)}_3 + 3 \text{O} + 3 \text{H}_2\text{O} \rightarrow 3 \text{HOC}8\text{H}{17} + \text{Al(OH)}_3 ]
Another method involves the dimerization of 1,3-butadiene with the addition of one molecule of water, catalyzed by palladium complexes, followed by hydrogenation .
Industrial Production Methods
The industrial production of octanol involves the hydrotreating of paraffin oil mixed with hydrogen at high temperature and pressure to reduce unsaturated hydrocarbons to saturated hydrocarbons. This is followed by an alcoholization reaction where the hydrotreated paraffin oil reacts with syngas (a mixture of hydrogen and carbon monoxide) under the action of a catalyst to produce octanol and water .
Chemical Reactions Analysis
Types of Reactions
Octanol undergoes various chemical reactions, including:
Oxidation: Octanol can be oxidized to octanoic acid.
Reduction: It can be reduced to octane.
Substitution: It can undergo substitution reactions to form esters, ethers, and halides.
Common Reagents and Conditions
Oxidation: Common oxidizing agents include potassium permanganate (KMnO₄) and chromium trioxide (CrO₃).
Reduction: Reducing agents such as lithium aluminium hydride (LiAlH₄) are used.
Substitution: Acid chlorides and alcohols are used to form esters, while halogenating agents like thionyl chloride (SOCl₂) are used to form halides.
Major Products
Oxidation: Octanoic acid.
Reduction: Octane.
Substitution: Octyl esters, octyl ethers, and octyl halides.
Scientific Research Applications
Octanol is widely used in scientific research due to its versatile properties:
Mechanism of Action
Octanol exerts its effects primarily through its interaction with lipid membranes. It disrupts membrane integrity, leading to increased permeability and potential cell lysis. This mechanism is particularly effective against microbial cells, where it induces apoptosis and disrupts metabolic pathways .
Comparison with Similar Compounds
Similar Compounds
1-Octanol: Another isomer of octanol with similar properties and uses.
Octanal: An aldehyde with the formula C₈H₁₆O, used in perfumery and flavoring.
Octanoic Acid: A carboxylic acid with the formula C₈H₁₆O₂, used in the production of esters and as a food additive.
Uniqueness
Octanol is unique due to its balanced hydrophilic and lipophilic properties, making it an excellent solvent and intermediate in various chemical reactions. Its ability to disrupt lipid membranes also makes it valuable in antimicrobial applications .
Properties
CAS No. |
78413-87-3 |
---|---|
Molecular Formula |
C7H5N3O6.C4H8N8O8.Al C11H13AlN11O14 |
Molecular Weight |
550.27 g/mol |
InChI |
InChI=1S/C7H5N3O6.C4H8N8O8.Al/c1-4-6(9(13)14)2-5(8(11)12)3-7(4)10(15)16;13-9(14)5-1-6(10(15)16)3-8(12(19)20)4-7(2-5)11(17)18;/h2-3H,1H3;1-4H2; |
InChI Key |
FTAFBCWHLFKBFJ-UHFFFAOYSA-N |
Canonical SMILES |
CC1=C(C=C(C=C1[N+](=O)[O-])[N+](=O)[O-])[N+](=O)[O-].C1N(CN(CN(CN1[N+](=O)[O-])[N+](=O)[O-])[N+](=O)[O-])[N+](=O)[O-].[Al] |
physical_description |
Octonal appears as a mixture of HMX, TNT, and powdered aluminum. An aluminum desensitized cyclotetramethylene tetranitranitramine. A colorless crystal sensitive to heat. Emits toxic oxides of nitrogen fumes when heated to decomposition. May explode under prolonged exposure to heat or fire. Primary hazard is blast of an instantaneous explosion, not flying projectiles or fragments. |
Origin of Product |
United States |
Disclaimer and Information on In-Vitro Research Products
Please be aware that all articles and product information presented on BenchChem are intended solely for informational purposes. The products available for purchase on BenchChem are specifically designed for in-vitro studies, which are conducted outside of living organisms. In-vitro studies, derived from the Latin term "in glass," involve experiments performed in controlled laboratory settings using cells or tissues. It is important to note that these products are not categorized as medicines or drugs, and they have not received approval from the FDA for the prevention, treatment, or cure of any medical condition, ailment, or disease. We must emphasize that any form of bodily introduction of these products into humans or animals is strictly prohibited by law. It is essential to adhere to these guidelines to ensure compliance with legal and ethical standards in research and experimentation.