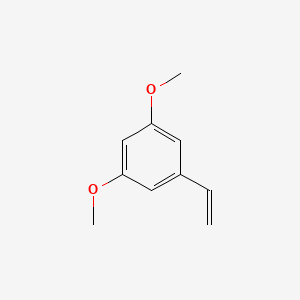
1-ethenyl-3,5-dimethoxybenzene
Overview
Description
1-Ethenyl-3,5-dimethoxybenzene is an organic compound with the molecular formula C10H12O2 . It is characterized by the presence of a benzene ring substituted with two methoxy groups at the 3 and 5 positions and an ethenyl group at the 1 position. This compound is known for its unique aromatic properties and is used in various chemical synthesis processes.
Preparation Methods
Synthetic Routes and Reaction Conditions: 1-Ethenyl-3,5-dimethoxybenzene can be synthesized through several methods. One common approach involves the reaction of 3,5-dimethoxybenzaldehyde with vinyl magnesium bromide in the presence of a catalyst such as copper(I) iodide . The reaction is typically carried out under an inert atmosphere to prevent oxidation and at a controlled temperature to ensure the desired product is obtained.
Industrial Production Methods: In an industrial setting, the production of this compound may involve the use of continuous flow reactors to maintain consistent reaction conditions and improve yield. The process may also include purification steps such as distillation or chromatography to isolate the compound from by-products and impurities.
Chemical Reactions Analysis
Types of Reactions: 1-Ethenyl-3,5-dimethoxybenzene undergoes various chemical reactions, including:
Oxidation: The ethenyl group can be oxidized to form corresponding aldehydes or carboxylic acids.
Reduction: The compound can be reduced to form 1-ethyl-3,5-dimethoxybenzene.
Substitution: Electrophilic aromatic substitution reactions can occur at the benzene ring, leading to the formation of various substituted derivatives.
Common Reagents and Conditions:
Oxidation: Reagents such as or are commonly used.
Reduction: Catalysts like or are employed.
Substitution: Reagents such as or in the presence of a Lewis acid catalyst like are used.
Major Products:
Oxidation: 3,5-dimethoxybenzaldehyde or 3,5-dimethoxybenzoic acid.
Reduction: 1-ethyl-3,5-dimethoxybenzene.
Substitution: Various halogenated derivatives depending on the substituent introduced.
Scientific Research Applications
1-Ethenyl-3,5-dimethoxybenzene has a wide range of applications in scientific research:
Chemistry: It is used as an intermediate in the synthesis of more complex organic molecules.
Biology: The compound is studied for its potential biological activities and interactions with biomolecules.
Medicine: Research is ongoing to explore its potential therapeutic properties and use in drug development.
Industry: It is used in the production of dyes, coatings, and other industrial chemicals.
Mechanism of Action
The mechanism by which 1-ethenyl-3,5-dimethoxybenzene exerts its effects involves interactions with various molecular targets. The ethenyl group can participate in π-π interactions with aromatic systems, while the methoxy groups can engage in hydrogen bonding and dipole-dipole interactions . These interactions can influence the compound’s reactivity and binding affinity to different targets.
Comparison with Similar Compounds
- 1-ethynyl-3,5-dimethoxybenzene
- 1-ethyl-3,5-dimethoxybenzene
- 1-bromo-3,5-dimethoxybenzene
Comparison: 1-Ethenyl-3,5-dimethoxybenzene is unique due to the presence of the ethenyl group, which imparts distinct reactivity compared to its ethynyl and ethyl analogs. The ethenyl group allows for additional reactions such as polymerization and cross-coupling, making it a versatile intermediate in organic synthesis.
Properties
IUPAC Name |
1-ethenyl-3,5-dimethoxybenzene | |
---|---|---|
Source | PubChem | |
URL | https://pubchem.ncbi.nlm.nih.gov | |
Description | Data deposited in or computed by PubChem | |
InChI |
InChI=1S/C10H12O2/c1-4-8-5-9(11-2)7-10(6-8)12-3/h4-7H,1H2,2-3H3 | |
Source | PubChem | |
URL | https://pubchem.ncbi.nlm.nih.gov | |
Description | Data deposited in or computed by PubChem | |
InChI Key |
YYDWZJCCIAMZIH-UHFFFAOYSA-N | |
Source | PubChem | |
URL | https://pubchem.ncbi.nlm.nih.gov | |
Description | Data deposited in or computed by PubChem | |
Canonical SMILES |
COC1=CC(=CC(=C1)C=C)OC | |
Source | PubChem | |
URL | https://pubchem.ncbi.nlm.nih.gov | |
Description | Data deposited in or computed by PubChem | |
Molecular Formula |
C10H12O2 | |
Source | PubChem | |
URL | https://pubchem.ncbi.nlm.nih.gov | |
Description | Data deposited in or computed by PubChem | |
DSSTOX Substance ID |
DTXSID70431125 | |
Record name | 3,5-dimethoxystyrene | |
Source | EPA DSSTox | |
URL | https://comptox.epa.gov/dashboard/DTXSID70431125 | |
Description | DSSTox provides a high quality public chemistry resource for supporting improved predictive toxicology. | |
Molecular Weight |
164.20 g/mol | |
Source | PubChem | |
URL | https://pubchem.ncbi.nlm.nih.gov | |
Description | Data deposited in or computed by PubChem | |
CAS No. |
40243-87-6 | |
Record name | 3,5-dimethoxystyrene | |
Source | EPA DSSTox | |
URL | https://comptox.epa.gov/dashboard/DTXSID70431125 | |
Description | DSSTox provides a high quality public chemistry resource for supporting improved predictive toxicology. | |
Synthesis routes and methods
Procedure details
Retrosynthesis Analysis
AI-Powered Synthesis Planning: Our tool employs the Template_relevance Pistachio, Template_relevance Bkms_metabolic, Template_relevance Pistachio_ringbreaker, Template_relevance Reaxys, Template_relevance Reaxys_biocatalysis model, leveraging a vast database of chemical reactions to predict feasible synthetic routes.
One-Step Synthesis Focus: Specifically designed for one-step synthesis, it provides concise and direct routes for your target compounds, streamlining the synthesis process.
Accurate Predictions: Utilizing the extensive PISTACHIO, BKMS_METABOLIC, PISTACHIO_RINGBREAKER, REAXYS, REAXYS_BIOCATALYSIS database, our tool offers high-accuracy predictions, reflecting the latest in chemical research and data.
Strategy Settings
Precursor scoring | Relevance Heuristic |
---|---|
Min. plausibility | 0.01 |
Model | Template_relevance |
Template Set | Pistachio/Bkms_metabolic/Pistachio_ringbreaker/Reaxys/Reaxys_biocatalysis |
Top-N result to add to graph | 6 |
Feasible Synthetic Routes
Q1: Can 3,5-Dimethoxystyrene be used to synthesize more complex molecules?
A2: Yes, 3,5-Dimethoxystyrene serves as a valuable building block in organic synthesis []. Researchers have successfully employed it in the synthesis of cyclic ketone derivatives, which hold potential as anti-inflammatory agents []. This highlights the versatility of 3,5-Dimethoxystyrene as a starting material for developing novel compounds with potential biological activities.
Q2: How does the configuration of trans-1,2-Bis(3,5-dimethoxyphenyl)ethene, a derivative of 3,5-Dimethoxystyrene, affect its molecular conformation?
A3: Interestingly, trans-1,2-Bis(3,5-dimethoxyphenyl)ethene, synthesized through Ru-catalyzed homo-olefin metathesis of 3,5-Dimethoxystyrene, exclusively forms the E-isomer []. Notably, within a single symmetric unit, two molecules of this compound adopt distinct conformations: s-syn-anti and all-s-anti. This observation underscores the influence of stereochemistry on the conformational preferences of molecules derived from 3,5-Dimethoxystyrene [].
Disclaimer and Information on In-Vitro Research Products
Please be aware that all articles and product information presented on BenchChem are intended solely for informational purposes. The products available for purchase on BenchChem are specifically designed for in-vitro studies, which are conducted outside of living organisms. In-vitro studies, derived from the Latin term "in glass," involve experiments performed in controlled laboratory settings using cells or tissues. It is important to note that these products are not categorized as medicines or drugs, and they have not received approval from the FDA for the prevention, treatment, or cure of any medical condition, ailment, or disease. We must emphasize that any form of bodily introduction of these products into humans or animals is strictly prohibited by law. It is essential to adhere to these guidelines to ensure compliance with legal and ethical standards in research and experimentation.