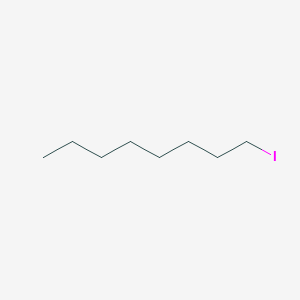
1-Iodooctane
Overview
Description
1-Iodooctane (CAS: 629-27-6) is a primary alkyl iodide with the molecular formula C₈H₁₇I. It is widely used in organic synthesis as an electrophilic alkylating agent due to its reactivity in substitution (e.g., SN2) and cross-coupling reactions. The compound is commercially available with high purity (typically >97–98%) from suppliers such as Aladdin, ENERGY Chemical, and Tokyo Chemical Industry, and is often used without further purification . Its linear structure (CH₃(CH₂)₆CH₂I) facilitates sterically accessible reactions at the iodine-bearing carbon, making it a preferred substrate in catalysis studies and pharmaceutical applications, such as the detection of alkyl halides in drug production via GC-MS .
Preparation Methods
1-Iodooctane can be synthesized through several methods. One common synthetic route involves the reaction of octanol with iodine and phosphorus trichloride. The reaction proceeds as follows:
C8H17OH+I2+PCl3→C8H17I+H3PO3+HCl
C8H17Br+NaI→C8H17I+NaBr
Industrial production methods typically involve the use of large-scale reactors and optimized conditions to maximize yield and purity .
Chemical Reactions Analysis
1-Iodooctane undergoes various chemical reactions, including:
Substitution Reactions: It can participate in nucleophilic substitution reactions, where the iodine atom is replaced by another nucleophile. For example, reacting with potassium cyanide to form octyl cyanide:
C8H17I+KCN→C8H17CN+KI
Reduction Reactions: It can be reduced to octane using reducing agents like lithium aluminum hydride (LiAlH4):
C8H17I+LiAlH4→C8H18+LiI+AlH3
Scientific Research Applications
1-Iodooctane is widely used in scientific research due to its versatility. Some applications include:
Organic Synthesis: It serves as an alkylating agent in the synthesis of various organic compounds.
Surface Chemistry: It is used in the formation of monolayers on silicon surfaces, studied by X-ray photoelectron spectroscopy.
Isotope Labeling: It reacts with lithium aluminum deuteride to form deuterated compounds, useful in NMR spectroscopy.
Catalysis: It is involved in the alkylation of nickel complexes, which are used in various catalytic processes.
Mechanism of Action
The mechanism of action of 1-iodooctane in chemical reactions typically involves the cleavage of the carbon-iodine bond, forming a reactive octyl cation or radical. This intermediate can then react with various nucleophiles or undergo further transformations. For example, in nucleophilic substitution reactions, the iodine atom is replaced by a nucleophile, forming a new carbon-nucleophile bond .
Comparison with Similar Compounds
Comparison with Similar Alkyl Iodides
Structural and Physical Properties
Alkyl iodides vary in chain length and molecular weight, influencing their physical properties and reactivity. Below is a comparison of 1-iodooctane with shorter- and longer-chain analogs:
Compound | CAS Number | Molecular Weight | Chain Length | Key Applications |
---|---|---|---|---|
1-Iodopropane | 107-08-4 | 169.99 g/mol | C3 | Small-scale alkylation reactions |
1-Iodohexane | 638-45-9 | 212.06 g/mol | C6 | Intermediate in organic synthesis |
This compound | 629-27-6 | 240.12 g/mol | C8 | Catalysis, GC-MS analysis |
1-Iododecane | 2050-77-3 | 268.18 g/mol | C10 | Polymer chemistry, surfactants |
1-Iodododecane | 4292-19-7 | 296.23 g/mol | C12 | Phase-transfer catalysis |
The longer carbon chain in this compound enhances its hydrophobicity compared to shorter analogs like 1-iodopropane, making it suitable for reactions in non-polar solvents. However, it retains sufficient volatility for GC-MS applications, unlike higher analogs like 1-iodododecane .
Reactivity in Substitution Reactions
This compound exhibits robust reactivity in SN2 reactions. For example, in the synthesis of octan-1-ol from this compound, a 92% yield is achieved using polymer-supported triphenylphosphine, outperforming analogs like iodocyclohexane (76% yield) . External electric fields (EEFs) further accelerate SN2 reactions involving this compound, reducing activation energy by ~6.0 kcal/mol under 1.0 V nm⁻¹, a more significant effect than solvent polarity alone .
Table 1: SN2 Reactivity of Selected Alkyl Iodides
Substrate | Reaction Conditions | Product Yield | Reference |
---|---|---|---|
This compound | EEF (1.0 V nm⁻¹), DMF | ~Quantitative | |
1-Iodohexane | Polar solvent (MeCN) | 85% | |
Iodocyclohexane | Polymer-supported PPh₃, RT | 76% |
Catalytic Coupling Reactions
This compound is extensively used in nickel- and iron-catalyzed couplings. For instance, in Kumada coupling with butylmagnesium chloride, this compound achieves yields comparable to 1-iododecane (~85%) using nickel pincer catalysts . Iron-catalyzed reductive coupling with nitroarenes produces N-octylaniline in 76% yield, demonstrating superior selectivity over secondary iodides .
Table 2: Cross-Coupling Performance of Alkyl Iodides
Stability and Handling
This compound requires stabilization with copper inhibitors or filtration through basic alumina to prevent decomposition, a step less critical for shorter-chain iodides like 1-iodopropane . Its shelf life and handling restrictions (e.g., controlled shipping) are comparable to 1-iodododecane but less stringent than highly volatile derivatives like ethyl iodide .
Research Findings and Data Tables
Kinetic Studies
Reaction order studies for this compound in nickel-catalyzed couplings show a linear dependence on concentration (0.25–0.55 M), with optimal yields achieved at 0.42 M :
Table 3: Rate Order Determination for this compound
Experiment | [this compound] (M) | Relative Rate |
---|---|---|
1 | 0.25 | 1.0 |
4 | 0.42 | 2.1 |
5 | 0.55 | 2.3 |
Biological Activity
1-Iodooctane, a halogenated aliphatic hydrocarbon, has garnered attention in various fields of research due to its unique biological properties and potential applications. This article delves into the biological activity of this compound, summarizing key findings from diverse studies, including toxicity profiles, antimicrobial effects, and its role in biochemical processes.
This compound is an alkyl iodide with the chemical formula C8H17I and a CAS number of 629-27-6. It is characterized by its hydrophobic nature and is often used in organic synthesis and as a reagent in various chemical reactions.
Toxicological Profile
The toxicological profile of this compound reveals significant insights into its biological effects:
- Acute Toxicity : The compound exhibits moderate acute toxicity, with reported intraperitoneal LD50 values of 1982 mg/kg for rats and 1416 mg/kg for mice, indicating a relatively high level of toxicity compared to other alkyl iodides .
- Chronic Effects : Long-term exposure can lead to cumulative health effects affecting multiple organ systems. Symptoms associated with exposure include central nervous system depression, headaches, nausea, and respiratory issues .
Antimicrobial Activity
Recent studies have explored the antimicrobial properties of this compound:
- Inhibition of Bacterial Growth : Research indicates that this compound possesses antimicrobial activity against various bacterial strains. For instance, it has been shown to inhibit growth in Staphylococcus aureus with a minimum inhibitory concentration (MIC) of 512 µg/mL . This suggests potential applications in developing antimicrobial agents.
Table 1: Antimicrobial Activity of this compound
Bacterial Strain | MIC (µg/mL) | MBC (µg/mL) |
---|---|---|
Staphylococcus aureus | 512 | >512 |
Escherichia coli | Not tested | Not tested |
Biochemical Interactions
This compound's interactions within biological systems are noteworthy:
- Enzyme Inhibition : Studies have indicated that this compound can inhibit iodotyrosine deiodinase (IYD), an enzyme critical for thyroid hormone synthesis. Inhibition of IYD leads to reduced iodide availability, which can disrupt normal thyroid function and development in organisms such as Xenopus laevis .
Case Study 1: Developmental Toxicity in Amphibians
A study evaluated the impact of IYD inhibition by this compound on the developmental stages of Xenopus laevis. The findings revealed that exposure to high concentrations resulted in delayed metamorphosis and altered thyroid hormone levels. This highlights the potential developmental risks associated with environmental exposure to halogenated compounds like this compound .
Case Study 2: Antimicrobial Efficacy
In another investigation, the antimicrobial efficacy of various alkyl iodides, including this compound, was assessed against pathogenic bacteria. The results demonstrated that while this compound exhibited some activity, its effectiveness varied significantly with chain length and structure modifications .
Q & A
Basic Research Questions
Q. How can the reaction order of 1-iodooctane be determined in nickel-catalyzed cross-coupling reactions?
To determine the reaction order, conduct kinetic experiments by varying the initial concentration of this compound while keeping other reactants constant. Use gas chromatography (GC) to monitor consumption rates and generate kinetic profiles. Plot logarithmic concentration versus time to identify the order (zero, first, or second). For example, experiments with 0.25–0.55 M this compound showed linear relationships in rate plots, confirming first-order dependence . Replicate trials three times to minimize experimental error.
Q. What are standard analytical protocols for quantifying this compound in reaction mixtures?
Use GC with flame ionization detection (FID) or mass spectrometry (GC-MS) for quantification. Internal standards (e.g., decane) improve accuracy. Calibrate with known concentrations of this compound and calculate conversion percentages using peak area ratios. For instance, GC-MS identified this compound and side products like 1-tert-butoxyoctane in catalytic reactions, with yields normalized to the alkyl halide .
Q. How are side reactions involving this compound characterized in catalytic systems?
Perform control experiments without substrates (e.g., alkynes) to isolate side products. Analyze via GC-MS and compare retention times with synthetic standards. For example, omitting oct-1-yne in Ni/CuI-catalyzed reactions revealed 1-tert-butoxyoctane (35% yield) and 1-octene as major side products, attributed to base-mediated elimination and nucleophilic substitution .
Advanced Research Questions
Q. How can contradictions in catalytic yield data be resolved when varying CuI concentrations?
Contradictions arise from competing roles of CuI as a co-catalyst and promoter of side reactions. Compare systems with/without CuI:
- With CuI (9 mol %): 39% coupling yield but 35% 1-tert-butoxyoctane due to CuI-mediated base activation .
- Without CuI: 6% coupling yield but minimal side products . Use statistical tools (e.g., error bars from triplicate trials) and mechanistic studies (e.g., radical trapping) to distinguish pathways.
Q. What is the impact of oriented external electric fields (EEFs) on SN2 reactions involving this compound?
Theoretical studies suggest EEFs aligned with the reaction axis lower activation barriers by stabilizing transition states. For this compound, a 1.0 V nm⁻¹ EEF reduced the energy barrier by ~6.0 kcal mol⁻¹, accelerating nucleophilic substitution. Experimental validation involves applying EEFs in electrochemical cells and monitoring kinetics via in situ spectroscopy .
Q. How can nickel catalysts be optimized for coupling this compound with alkynes?
Screen ligands, bases, and solvents systematically. For example:
- Ligand effects: Complex 25 (bipyridine-type) achieved 74% yield in DMF, outperforming other ligands .
- Solvent effects: Polar solvents (DMF) enhance ion dissociation, improving reactivity over non-polar solvents (dioxane) .
- Base selection: LiOtBu outperformed Cs₂CO₃ due to stronger activation of the alkyne .
Q. How do environmental factors influence the stability and reactivity of this compound in marine systems?
In Laminaria digitata algae, this compound contributes to aroma via oxidative stress pathways. Study its stability under thermal/mechanical treatments using headspace GC-MS. For instance, this compound levels remained stable in heated samples, suggesting resistance to degradation .
Q. Data Analysis & Interpretation
Q. How should researchers interpret rate constant variations in this compound coupling reactions?
Rate constants (kcat) vary with reactant concentrations and catalyst loading. For example:
- Varying [this compound]: kcat = 1.8 × 10⁻³ s⁻¹ (10% error) .
- Varying [CuI]: kcat = 1.5 × 10⁻³ s⁻¹ (10% error) . Use error propagation analysis and Arrhenius plots to distinguish kinetic regimes (e.g., rate-limiting oxidative addition vs. transmetalation).
Q. What methodologies address discrepancies in mass balance during this compound reactions?
Discrepancies arise from volatile byproducts or unaccounted intermediates. Employ quantitative NMR or isotopic labeling (e.g., ¹³C-1-iodooctane) to track carbon flow. In one study, 80% mass recovery was achieved by accounting for 1-octene (30%) and 1-tert-butoxyoctane (20%) .
Q. Experimental Design
Q. What controls are essential for reproducibility in this compound cross-coupling studies?
Include:
- Blank reactions (no catalyst/base) to assess background reactivity.
- Internal standards (e.g., tetradecane) for GC quantification.
- Triplicate trials to calculate mean yields and standard deviations .
Properties
IUPAC Name |
1-iodooctane | |
---|---|---|
Source | PubChem | |
URL | https://pubchem.ncbi.nlm.nih.gov | |
Description | Data deposited in or computed by PubChem | |
InChI |
InChI=1S/C8H17I/c1-2-3-4-5-6-7-8-9/h2-8H2,1H3 | |
Source | PubChem | |
URL | https://pubchem.ncbi.nlm.nih.gov | |
Description | Data deposited in or computed by PubChem | |
InChI Key |
UWLHSHAHTBJTBA-UHFFFAOYSA-N | |
Source | PubChem | |
URL | https://pubchem.ncbi.nlm.nih.gov | |
Description | Data deposited in or computed by PubChem | |
Canonical SMILES |
CCCCCCCCI | |
Source | PubChem | |
URL | https://pubchem.ncbi.nlm.nih.gov | |
Description | Data deposited in or computed by PubChem | |
Molecular Formula |
C8H17I | |
Source | PubChem | |
URL | https://pubchem.ncbi.nlm.nih.gov | |
Description | Data deposited in or computed by PubChem | |
DSSTOX Substance ID |
DTXSID4060868 | |
Record name | Octane, 1-iodo- | |
Source | EPA DSSTox | |
URL | https://comptox.epa.gov/dashboard/DTXSID4060868 | |
Description | DSSTox provides a high quality public chemistry resource for supporting improved predictive toxicology. | |
Molecular Weight |
240.12 g/mol | |
Source | PubChem | |
URL | https://pubchem.ncbi.nlm.nih.gov | |
Description | Data deposited in or computed by PubChem | |
Physical Description |
Clear, light yellow liquid; [Aldrich MSDS] | |
Record name | 1-Iodooctane | |
Source | Haz-Map, Information on Hazardous Chemicals and Occupational Diseases | |
URL | https://haz-map.com/Agents/9621 | |
Description | Haz-Map® is an occupational health database designed for health and safety professionals and for consumers seeking information about the adverse effects of workplace exposures to chemical and biological agents. | |
Explanation | Copyright (c) 2022 Haz-Map(R). All rights reserved. Unless otherwise indicated, all materials from Haz-Map are copyrighted by Haz-Map(R). No part of these materials, either text or image may be used for any purpose other than for personal use. Therefore, reproduction, modification, storage in a retrieval system or retransmission, in any form or by any means, electronic, mechanical or otherwise, for reasons other than personal use, is strictly prohibited without prior written permission. | |
CAS No. |
629-27-6 | |
Record name | 1-Iodooctane | |
Source | CAS Common Chemistry | |
URL | https://commonchemistry.cas.org/detail?cas_rn=629-27-6 | |
Description | CAS Common Chemistry is an open community resource for accessing chemical information. Nearly 500,000 chemical substances from CAS REGISTRY cover areas of community interest, including common and frequently regulated chemicals, and those relevant to high school and undergraduate chemistry classes. This chemical information, curated by our expert scientists, is provided in alignment with our mission as a division of the American Chemical Society. | |
Explanation | The data from CAS Common Chemistry is provided under a CC-BY-NC 4.0 license, unless otherwise stated. | |
Record name | Octane, 1-iodo- | |
Source | ChemIDplus | |
URL | https://pubchem.ncbi.nlm.nih.gov/substance/?source=chemidplus&sourceid=0000629276 | |
Description | ChemIDplus is a free, web search system that provides access to the structure and nomenclature authority files used for the identification of chemical substances cited in National Library of Medicine (NLM) databases, including the TOXNET system. | |
Record name | Octane, 1-iodo- | |
Source | EPA Chemicals under the TSCA | |
URL | https://www.epa.gov/chemicals-under-tsca | |
Description | EPA Chemicals under the Toxic Substances Control Act (TSCA) collection contains information on chemicals and their regulations under TSCA, including non-confidential content from the TSCA Chemical Substance Inventory and Chemical Data Reporting. | |
Record name | Octane, 1-iodo- | |
Source | EPA DSSTox | |
URL | https://comptox.epa.gov/dashboard/DTXSID4060868 | |
Description | DSSTox provides a high quality public chemistry resource for supporting improved predictive toxicology. | |
Record name | 1-iodooctane | |
Source | European Chemicals Agency (ECHA) | |
URL | https://echa.europa.eu/substance-information/-/substanceinfo/100.010.077 | |
Description | The European Chemicals Agency (ECHA) is an agency of the European Union which is the driving force among regulatory authorities in implementing the EU's groundbreaking chemicals legislation for the benefit of human health and the environment as well as for innovation and competitiveness. | |
Explanation | Use of the information, documents and data from the ECHA website is subject to the terms and conditions of this Legal Notice, and subject to other binding limitations provided for under applicable law, the information, documents and data made available on the ECHA website may be reproduced, distributed and/or used, totally or in part, for non-commercial purposes provided that ECHA is acknowledged as the source: "Source: European Chemicals Agency, http://echa.europa.eu/". Such acknowledgement must be included in each copy of the material. ECHA permits and encourages organisations and individuals to create links to the ECHA website under the following cumulative conditions: Links can only be made to webpages that provide a link to the Legal Notice page. | |
Synthesis routes and methods I
Procedure details
Synthesis routes and methods II
Procedure details
Retrosynthesis Analysis
AI-Powered Synthesis Planning: Our tool employs the Template_relevance Pistachio, Template_relevance Bkms_metabolic, Template_relevance Pistachio_ringbreaker, Template_relevance Reaxys, Template_relevance Reaxys_biocatalysis model, leveraging a vast database of chemical reactions to predict feasible synthetic routes.
One-Step Synthesis Focus: Specifically designed for one-step synthesis, it provides concise and direct routes for your target compounds, streamlining the synthesis process.
Accurate Predictions: Utilizing the extensive PISTACHIO, BKMS_METABOLIC, PISTACHIO_RINGBREAKER, REAXYS, REAXYS_BIOCATALYSIS database, our tool offers high-accuracy predictions, reflecting the latest in chemical research and data.
Strategy Settings
Precursor scoring | Relevance Heuristic |
---|---|
Min. plausibility | 0.01 |
Model | Template_relevance |
Template Set | Pistachio/Bkms_metabolic/Pistachio_ringbreaker/Reaxys/Reaxys_biocatalysis |
Top-N result to add to graph | 6 |
Feasible Synthetic Routes
Disclaimer and Information on In-Vitro Research Products
Please be aware that all articles and product information presented on BenchChem are intended solely for informational purposes. The products available for purchase on BenchChem are specifically designed for in-vitro studies, which are conducted outside of living organisms. In-vitro studies, derived from the Latin term "in glass," involve experiments performed in controlled laboratory settings using cells or tissues. It is important to note that these products are not categorized as medicines or drugs, and they have not received approval from the FDA for the prevention, treatment, or cure of any medical condition, ailment, or disease. We must emphasize that any form of bodily introduction of these products into humans or animals is strictly prohibited by law. It is essential to adhere to these guidelines to ensure compliance with legal and ethical standards in research and experimentation.