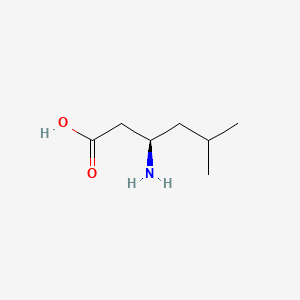
(R)-3-Amino-5-methyl-hexanoic acid
Overview
Description
®-3-Amino-5-methyl-hexanoic acid is an organic compound that belongs to the class of amino acids. It is characterized by the presence of an amino group (-NH2) and a carboxylic acid group (-COOH) attached to a hexane backbone with a methyl group at the fifth position. This compound is chiral, meaning it has a non-superimposable mirror image, and the ® designation indicates the specific configuration of the molecule.
Preparation Methods
Synthetic Routes and Reaction Conditions
The synthesis of ®-3-Amino-5-methyl-hexanoic acid can be achieved through several methods:
Amidomalonate Synthesis: This method involves the alkylation of diethyl acetamidomalonate with a suitable alkyl halide, followed by hydrolysis and decarboxylation to yield the desired amino acid.
Reductive Amination: This approach involves the reductive amination of an α-keto acid with ammonia and a reducing agent such as sodium borohydride (NaBH4).
Industrial Production Methods
In industrial settings, the production of ®-3-Amino-5-methyl-hexanoic acid often involves the use of chiral catalysts to ensure the desired enantiomer is obtained with high purity. Enantioselective hydrogenation of a suitable precursor using a chiral rhodium catalyst is one such method .
Chemical Reactions Analysis
Types of Reactions
®-3-Amino-5-methyl-hexanoic acid undergoes various chemical reactions, including:
Oxidation: The amino group can be oxidized to form corresponding oximes or nitriles.
Reduction: The carboxylic acid group can be reduced to an alcohol using reducing agents like lithium aluminum hydride (LiAlH4).
Substitution: The amino group can participate in nucleophilic substitution reactions to form amides or other derivatives.
Common Reagents and Conditions
Oxidation: Reagents such as potassium permanganate (KMnO4) or chromium trioxide (CrO3) are commonly used.
Reduction: Lithium aluminum hydride (LiAlH4) or sodium borohydride (NaBH4) are typical reducing agents.
Substitution: Ammonia (NH3) or primary amines are used for nucleophilic substitution reactions.
Major Products Formed
Oxidation: Oximes, nitriles
Reduction: Alcohols
Substitution: Amides, substituted amines
Scientific Research Applications
®-3-Amino-5-methyl-hexanoic acid has a wide range of applications in scientific research:
Chemistry: It is used as a building block in the synthesis of more complex molecules.
Biology: It serves as a precursor for the synthesis of peptides and proteins.
Medicine: It is investigated for its potential therapeutic effects, including its role in neurotransmission and as a potential treatment for certain neurological disorders.
Mechanism of Action
The mechanism of action of ®-3-Amino-5-methyl-hexanoic acid involves its interaction with specific molecular targets and pathways:
Molecular Targets: It interacts with enzymes and receptors involved in neurotransmission.
Pathways Involved: It modulates the activity of neurotransmitters, potentially influencing synaptic transmission and neuronal communication.
Comparison with Similar Compounds
Similar Compounds
L-Leucine: An essential amino acid with a similar structure but different side chain.
L-Isoleucine: Another essential amino acid with a branched-chain structure.
L-Valine: A branched-chain amino acid with a similar backbone but different side chain.
Uniqueness
®-3-Amino-5-methyl-hexanoic acid is unique due to its specific chiral configuration and the presence of a methyl group at the fifth position, which distinguishes it from other amino acids and imparts unique chemical and biological properties .
Properties
IUPAC Name |
(3R)-3-amino-5-methylhexanoic acid | |
---|---|---|
Source | PubChem | |
URL | https://pubchem.ncbi.nlm.nih.gov | |
Description | Data deposited in or computed by PubChem | |
InChI |
InChI=1S/C7H15NO2/c1-5(2)3-6(8)4-7(9)10/h5-6H,3-4,8H2,1-2H3,(H,9,10)/t6-/m1/s1 | |
Source | PubChem | |
URL | https://pubchem.ncbi.nlm.nih.gov | |
Description | Data deposited in or computed by PubChem | |
InChI Key |
MLYMSIKVLAPCAK-ZCFIWIBFSA-N | |
Source | PubChem | |
URL | https://pubchem.ncbi.nlm.nih.gov | |
Description | Data deposited in or computed by PubChem | |
Canonical SMILES |
CC(C)CC(CC(=O)O)N | |
Source | PubChem | |
URL | https://pubchem.ncbi.nlm.nih.gov | |
Description | Data deposited in or computed by PubChem | |
Isomeric SMILES |
CC(C)C[C@H](CC(=O)O)N | |
Source | PubChem | |
URL | https://pubchem.ncbi.nlm.nih.gov | |
Description | Data deposited in or computed by PubChem | |
Molecular Formula |
C7H15NO2 | |
Source | PubChem | |
URL | https://pubchem.ncbi.nlm.nih.gov | |
Description | Data deposited in or computed by PubChem | |
DSSTOX Substance ID |
DTXSID80426163 | |
Record name | (R)-3-Amino-5-methyl-hexanoic acid | |
Source | EPA DSSTox | |
URL | https://comptox.epa.gov/dashboard/DTXSID80426163 | |
Description | DSSTox provides a high quality public chemistry resource for supporting improved predictive toxicology. | |
Molecular Weight |
145.20 g/mol | |
Source | PubChem | |
URL | https://pubchem.ncbi.nlm.nih.gov | |
Description | Data deposited in or computed by PubChem | |
CAS No. |
91298-67-8 | |
Record name | (R)-3-Amino-5-methyl-hexanoic acid | |
Source | EPA DSSTox | |
URL | https://comptox.epa.gov/dashboard/DTXSID80426163 | |
Description | DSSTox provides a high quality public chemistry resource for supporting improved predictive toxicology. | |
Record name | 91298-67-8 | |
Source | European Chemicals Agency (ECHA) | |
URL | https://echa.europa.eu/information-on-chemicals | |
Description | The European Chemicals Agency (ECHA) is an agency of the European Union which is the driving force among regulatory authorities in implementing the EU's groundbreaking chemicals legislation for the benefit of human health and the environment as well as for innovation and competitiveness. | |
Explanation | Use of the information, documents and data from the ECHA website is subject to the terms and conditions of this Legal Notice, and subject to other binding limitations provided for under applicable law, the information, documents and data made available on the ECHA website may be reproduced, distributed and/or used, totally or in part, for non-commercial purposes provided that ECHA is acknowledged as the source: "Source: European Chemicals Agency, http://echa.europa.eu/". Such acknowledgement must be included in each copy of the material. ECHA permits and encourages organisations and individuals to create links to the ECHA website under the following cumulative conditions: Links can only be made to webpages that provide a link to the Legal Notice page. | |
Synthesis routes and methods
Procedure details
Retrosynthesis Analysis
AI-Powered Synthesis Planning: Our tool employs the Template_relevance Pistachio, Template_relevance Bkms_metabolic, Template_relevance Pistachio_ringbreaker, Template_relevance Reaxys, Template_relevance Reaxys_biocatalysis model, leveraging a vast database of chemical reactions to predict feasible synthetic routes.
One-Step Synthesis Focus: Specifically designed for one-step synthesis, it provides concise and direct routes for your target compounds, streamlining the synthesis process.
Accurate Predictions: Utilizing the extensive PISTACHIO, BKMS_METABOLIC, PISTACHIO_RINGBREAKER, REAXYS, REAXYS_BIOCATALYSIS database, our tool offers high-accuracy predictions, reflecting the latest in chemical research and data.
Strategy Settings
Precursor scoring | Relevance Heuristic |
---|---|
Min. plausibility | 0.01 |
Model | Template_relevance |
Template Set | Pistachio/Bkms_metabolic/Pistachio_ringbreaker/Reaxys/Reaxys_biocatalysis |
Top-N result to add to graph | 6 |
Feasible Synthetic Routes
Disclaimer and Information on In-Vitro Research Products
Please be aware that all articles and product information presented on BenchChem are intended solely for informational purposes. The products available for purchase on BenchChem are specifically designed for in-vitro studies, which are conducted outside of living organisms. In-vitro studies, derived from the Latin term "in glass," involve experiments performed in controlled laboratory settings using cells or tissues. It is important to note that these products are not categorized as medicines or drugs, and they have not received approval from the FDA for the prevention, treatment, or cure of any medical condition, ailment, or disease. We must emphasize that any form of bodily introduction of these products into humans or animals is strictly prohibited by law. It is essential to adhere to these guidelines to ensure compliance with legal and ethical standards in research and experimentation.