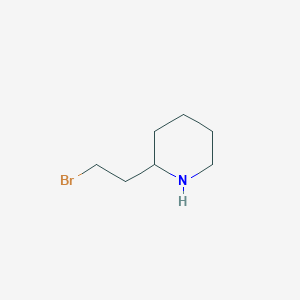
2-(2-Bromoethyl)piperidine
Overview
Description
2-(2-Bromoethyl)piperidine is a useful research compound. Its molecular formula is C7H14BrN and its molecular weight is 192.1 g/mol. The purity is usually 95%.
BenchChem offers high-quality this compound suitable for many research applications. Different packaging options are available to accommodate customers' requirements. Please inquire for more information about this compound including the price, delivery time, and more detailed information at info@benchchem.com.
Scientific Research Applications
Synthesis of Complex Molecules
2-(2-Bromoethyl)piperidine has been utilized in the synthesis of complex molecules. D’hooghe et al. (2009) demonstrated its application in the synthesis of 2-(methoxycarbonyl)indolizidine, a novel compound, through conjugate addition to alkyl acrylates (D’hooghe et al., 2009). Similarly, Freund and Mederski (2000) developed a method to synthesize spiro[indole-3,4′-piperidin]-2-ones, starting from 2-bromoaniline and 1-[(tert-butoxy)carbonyl]piperidine-4-carboxylic acid, emphasizing the compound's versatility in creating spiropiperidines (Freund & Mederski, 2000).
Pharmaceutical Building Blocks
Lennox et al. (2018) reported an electrochemical method for the cyanation of secondary piperidines, highlighting this compound's potential as a pharmaceutical building block. This process allows for the synthesis of unnatural amino acids, further demonstrating its relevance in pharmaceutical research (Lennox et al., 2018).
Enantioselective Synthesis
Perdicchia et al. (2015) utilized 2-piperidineethanol, a derivative of this compound, for the enantioselective synthesis of various natural and synthetic compounds. This highlights its role in facilitating stereochemically precise syntheses, essential for creating biologically active molecules (Perdicchia et al., 2015).
Stereoselective Synthesis
McCall and Comins (2009) explored the stereoselective synthesis of alkylamine derivatives through von Braun ring opening of chiral piperidines, indicating the significance of this compound in creating diverse chemical structures with multiple chiral centers (McCall & Comins, 2009).
Radical Cyclization
Gandon et al. (2006) reported a novel approach to synthesizing 2,4-disubstituted piperidines involving the radical cyclization of this compound derivatives. This method underscores its utility in generating structurally complex piperidines (Gandon et al., 2006).
Future Directions
Mechanism of Action
Target of Action
2-(2-Bromoethyl)piperidine is a derivative of piperidine, a heterocyclic moiety . Piperidine and its derivatives have been observed to have therapeutic properties, including anticancer potential . They act as potential clinical agents against various types of cancers, such as breast cancer, prostate cancer, colon cancer, lung cancer, and ovarian cancer .
Mode of Action
Piperidine derivatives have been observed to regulate several crucial signaling pathways essential for the establishment of cancers . These include STAT-3, NF-κB, PI3k/Aκt, JNK/p38-MAPK, TGF-ß/SMAD, Smac/DIABLO, p-IκB, and others . These phytochemicals lead to inhibition of cell migration and help in cell cycle arrest to inhibit the survivability of cancer cells .
Biochemical Pathways
Piperidine and its derivatives, including this compound, have been observed to affect multiple signaling pathways. These include Akt/mTOR/MMP-9, 5′-AMP-activated protein kinase-activated NLR family pyrin domain containing-3 inflammasome, voltage-gated K+ current, PKCα/ERK1/2, NF-κB/AP-1/MMP-9, Wnt/β-catenin, JNK/P38 MAPK, and gut microbiota .
Pharmacokinetics
Piperine, a related compound, has been observed to alter gastrointestinal disorders, drug-metabolizing enzymes, and the bioavailability of several drugs . This suggests that this compound may have similar effects on absorption, distribution, metabolism, and excretion (ADME) properties.
Result of Action
Piperidine and its derivatives have been observed to inhibit cell migration and help in cell cycle arrest, thereby inhibiting the survivability of cancer cells .
Biochemical Analysis
Biochemical Properties
2-(2-Bromoethyl)piperidine plays a significant role in various biochemical reactions. It interacts with several enzymes, proteins, and other biomolecules. For instance, it has been observed to interact with cytochrome P450 enzymes, which are crucial for drug metabolism. The interaction involves the activation of the N-H bond, leading to the formation of a carbon-centered radical intermediate . This interaction is essential for the compound’s role in biochemical processes.
Cellular Effects
This compound has notable effects on various types of cells and cellular processes. It influences cell function by affecting cell signaling pathways, gene expression, and cellular metabolism. For example, it has been shown to modulate the activity of certain signaling pathways, leading to changes in gene expression and metabolic flux . These effects are crucial for understanding the compound’s potential therapeutic applications.
Molecular Mechanism
The molecular mechanism of this compound involves several key interactions at the molecular level. The compound exerts its effects through binding interactions with biomolecules, enzyme inhibition or activation, and changes in gene expression. Specifically, it interacts with cytochrome P450 enzymes, leading to the formation of a radical intermediate that undergoes further reactions to produce the desired biochemical effects . This mechanism is vital for the compound’s role in various biochemical processes.
Temporal Effects in Laboratory Settings
In laboratory settings, the effects of this compound change over time. The compound’s stability and degradation are critical factors that influence its long-term effects on cellular function. Studies have shown that the compound remains stable under specific conditions, but its degradation can lead to changes in its biochemical activity . These temporal effects are essential for understanding the compound’s behavior in laboratory experiments.
Dosage Effects in Animal Models
The effects of this compound vary with different dosages in animal models. At lower doses, the compound exhibits beneficial effects, such as modulating enzyme activity and gene expression. At higher doses, it can cause toxic or adverse effects, including respiratory paralysis and organ dysfunction . Understanding these dosage effects is crucial for determining the compound’s therapeutic potential and safety.
Metabolic Pathways
This compound is involved in several metabolic pathways. It interacts with enzymes such as cytochrome P450, which play a crucial role in its metabolism. The compound undergoes ring contraction mediated by cytochrome P450 enzymes, leading to the formation of a ring-contracted pyrrolidine product . These metabolic pathways are essential for understanding the compound’s biochemical activity and potential therapeutic applications.
Transport and Distribution
The transport and distribution of this compound within cells and tissues are critical for its biochemical activity. The compound interacts with specific transporters and binding proteins that facilitate its movement across cellular membranes . These interactions influence the compound’s localization and accumulation within different tissues, affecting its overall activity and function.
Subcellular Localization
This compound exhibits specific subcellular localization patterns that influence its activity and function. The compound is directed to specific compartments or organelles within the cell through targeting signals and post-translational modifications . These localization patterns are crucial for understanding the compound’s role in various cellular processes and its potential therapeutic applications.
Properties
IUPAC Name |
2-(2-bromoethyl)piperidine | |
---|---|---|
Source | PubChem | |
URL | https://pubchem.ncbi.nlm.nih.gov | |
Description | Data deposited in or computed by PubChem | |
InChI |
InChI=1S/C7H14BrN/c8-5-4-7-3-1-2-6-9-7/h7,9H,1-6H2 | |
Source | PubChem | |
URL | https://pubchem.ncbi.nlm.nih.gov | |
Description | Data deposited in or computed by PubChem | |
InChI Key |
UISQNTXFRUUKBZ-UHFFFAOYSA-N | |
Source | PubChem | |
URL | https://pubchem.ncbi.nlm.nih.gov | |
Description | Data deposited in or computed by PubChem | |
Canonical SMILES |
C1CCNC(C1)CCBr | |
Source | PubChem | |
URL | https://pubchem.ncbi.nlm.nih.gov | |
Description | Data deposited in or computed by PubChem | |
Molecular Formula |
C7H14BrN | |
Source | PubChem | |
URL | https://pubchem.ncbi.nlm.nih.gov | |
Description | Data deposited in or computed by PubChem | |
DSSTOX Substance ID |
DTXSID60424682 | |
Record name | 2-(2-bromoethyl)piperidine | |
Source | EPA DSSTox | |
URL | https://comptox.epa.gov/dashboard/DTXSID60424682 | |
Description | DSSTox provides a high quality public chemistry resource for supporting improved predictive toxicology. | |
Molecular Weight |
192.10 g/mol | |
Source | PubChem | |
URL | https://pubchem.ncbi.nlm.nih.gov | |
Description | Data deposited in or computed by PubChem | |
CAS No. |
731742-05-5 | |
Record name | 2-(2-bromoethyl)piperidine | |
Source | EPA DSSTox | |
URL | https://comptox.epa.gov/dashboard/DTXSID60424682 | |
Description | DSSTox provides a high quality public chemistry resource for supporting improved predictive toxicology. | |
Retrosynthesis Analysis
AI-Powered Synthesis Planning: Our tool employs the Template_relevance Pistachio, Template_relevance Bkms_metabolic, Template_relevance Pistachio_ringbreaker, Template_relevance Reaxys, Template_relevance Reaxys_biocatalysis model, leveraging a vast database of chemical reactions to predict feasible synthetic routes.
One-Step Synthesis Focus: Specifically designed for one-step synthesis, it provides concise and direct routes for your target compounds, streamlining the synthesis process.
Accurate Predictions: Utilizing the extensive PISTACHIO, BKMS_METABOLIC, PISTACHIO_RINGBREAKER, REAXYS, REAXYS_BIOCATALYSIS database, our tool offers high-accuracy predictions, reflecting the latest in chemical research and data.
Strategy Settings
Precursor scoring | Relevance Heuristic |
---|---|
Min. plausibility | 0.01 |
Model | Template_relevance |
Template Set | Pistachio/Bkms_metabolic/Pistachio_ringbreaker/Reaxys/Reaxys_biocatalysis |
Top-N result to add to graph | 6 |
Feasible Synthetic Routes
Q1: What are the main applications of 2-(2-Bromoethyl)piperidine in organic synthesis?
A1: this compound serves as a valuable reagent in synthesizing complex heterocyclic compounds. Its reactivity stems from the presence of both a piperidine ring and a bromoethyl group. For instance, it acts as an alkylating agent in reactions with potassium salts of enol ethers. [, ] This property proves beneficial in constructing fused ring systems, particularly those relevant to medicinal chemistry, as demonstrated in the synthesis of 8-aza-D-homo-18-norestrone methyl ether. [] Additionally, the compound participates in conjugate addition reactions with alkyl acrylates, ultimately leading to substituted piperidine derivatives. [] This approach offers a route to indolizidine frameworks, which hold significance in pharmaceutical research.
Q2: Can you provide an example of a specific synthetic application of this compound?
A2: One notable application involves the synthesis of 2-(methoxycarbonyl)indolizidine. [] Researchers achieved this by reacting this compound with methyl acrylate, followed by a cyclization step facilitated by lithium diisopropylamide. This synthetic route highlights the versatility of this compound in constructing complex heterocycles.
Q3: What structural features of this compound make it valuable in these synthetic applications?
A3: The compound's utility arises from the combination of the nucleophilic nitrogen atom within the piperidine ring and the electrophilic carbon atom bonded to the bromine atom in the bromoethyl group. This dual reactivity allows it to participate in various reactions, leading to the formation of new carbon-nitrogen bonds and the creation of diverse cyclic structures. [, ]
Disclaimer and Information on In-Vitro Research Products
Please be aware that all articles and product information presented on BenchChem are intended solely for informational purposes. The products available for purchase on BenchChem are specifically designed for in-vitro studies, which are conducted outside of living organisms. In-vitro studies, derived from the Latin term "in glass," involve experiments performed in controlled laboratory settings using cells or tissues. It is important to note that these products are not categorized as medicines or drugs, and they have not received approval from the FDA for the prevention, treatment, or cure of any medical condition, ailment, or disease. We must emphasize that any form of bodily introduction of these products into humans or animals is strictly prohibited by law. It is essential to adhere to these guidelines to ensure compliance with legal and ethical standards in research and experimentation.