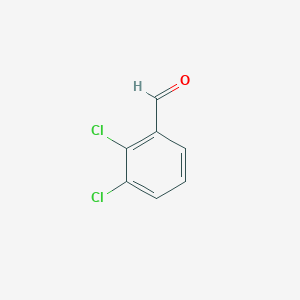
2,3-Dichlorobenzaldehyde
Overview
Description
2,3-Dichlorobenzaldehyde is a chemical compound with the formula C7H4Cl2O. It has a molecular weight of 175.01 .
Synthesis Analysis
There are several methods for synthesizing 2,3-Dichlorobenzaldehyde. One method involves using 2,3-dichlorotoluene as a raw material, bromine as an auxiliary material, and azodiisobutyronitrile as a catalyst . Another method involves using 2,3-dichloroaniline hydrochloride as a raw material to prepare a diazo salt, which then reacts with formaldoxime .Molecular Structure Analysis
The molecular structure of 2,3-Dichlorobenzaldehyde consists of a benzene ring with two chlorine atoms and one aldehyde group attached to it .Physical And Chemical Properties Analysis
2,3-Dichlorobenzaldehyde appears as white chunks. It is insoluble in water, has a melting point of 64°C, a boiling point of 243°C, and a density of 1.33 g/cm3 at 100°C .Scientific Research Applications
Synthesis of Antihypertensive Agents
2,3-Dichlorobenzaldehyde is a key intermediate in the synthesis of felodipine , a medication used to treat high blood pressure. The compound’s structure, with three groups in adjacent positions, presents a challenge due to steric hindrance, making its synthesis complex and intriguing for researchers .
Preparation of Schiff Bases
This compound is utilized in the preparation of Schiff bases , which are typically formed by the condensation of primary amines with aldehydes. These bases have various applications, including their use as dyes and pigments, and in the development of various pharmaceuticals .
Biginelli Condensation
In the realm of organic synthesis, 2,3-Dichlorobenzaldehyde is employed in the Biginelli reaction . This is a multicomponent chemical reaction that creates 3,4-dihydropyrimidin-2(1H)-ones, which are valuable compounds in medicinal chemistry .
Organic Synthesis Techniques
Innovative synthesis techniques using 2,3-Dichlorobenzaldehyde are being developed to improve yields and reduce environmental impact. These methods are crucial for the large-scale production of high-purity compounds required in pharmaceuticals and other industries .
Safety and Hazards
Mechanism of Action
Target of Action
It has been used in the preparation of various compounds, suggesting that it may interact with a variety of molecular targets .
Mode of Action
It has been used in the synthesis of compounds such as 4-(2,3-dichlorobenzylideneamino)-1,5-dimethyl-2-phenyl-1h-pyrazol-3(2h)-one and ethyl 4-(2,3-dichlorophenyl)-1,6-dimethyl-2-oxo-1,2,3,4-tetrahydropyrimidine-5-carboxylate . These reactions suggest that 2,3-Dichlorobenzaldehyde may interact with its targets through the formation of covalent bonds, leading to changes in the targets’ structure and function .
Pharmacokinetics
It is suggested that the compound has high gastrointestinal absorption and is bbb permeant . It is also suggested to be a CYP1A2 inhibitor . The compound’s lipophilicity (Log Po/w) is reported to be 1.77 (iLOGP) and 2.96 (XLOGP3) . These properties may impact the bioavailability of 2,3-Dichlorobenzaldehyde.
Result of Action
It is known to cause skin burns, eye damage, and respiratory irritation .
Action Environment
Environmental factors can influence the action, efficacy, and stability of 2,3-Dichlorobenzaldehyde. For instance, it has been found to be toxic to aquatic algae and cyanobacteria . Furthermore, it is recommended to be stored in a dark place, sealed in dry, at room temperature , indicating that light, moisture, and temperature could affect its stability and efficacy.
properties
IUPAC Name |
2,3-dichlorobenzaldehyde | |
---|---|---|
Source | PubChem | |
URL | https://pubchem.ncbi.nlm.nih.gov | |
Description | Data deposited in or computed by PubChem | |
InChI |
InChI=1S/C7H4Cl2O/c8-6-3-1-2-5(4-10)7(6)9/h1-4H | |
Source | PubChem | |
URL | https://pubchem.ncbi.nlm.nih.gov | |
Description | Data deposited in or computed by PubChem | |
InChI Key |
LLMLNAVBOAMOEE-UHFFFAOYSA-N | |
Source | PubChem | |
URL | https://pubchem.ncbi.nlm.nih.gov | |
Description | Data deposited in or computed by PubChem | |
Canonical SMILES |
C1=CC(=C(C(=C1)Cl)Cl)C=O | |
Source | PubChem | |
URL | https://pubchem.ncbi.nlm.nih.gov | |
Description | Data deposited in or computed by PubChem | |
Molecular Formula |
C7H4Cl2O | |
Source | PubChem | |
URL | https://pubchem.ncbi.nlm.nih.gov | |
Description | Data deposited in or computed by PubChem | |
DSSTOX Substance ID |
DTXSID7064239 | |
Record name | 2,3-Dichlorobenzaldehyde | |
Source | EPA DSSTox | |
URL | https://comptox.epa.gov/dashboard/DTXSID7064239 | |
Description | DSSTox provides a high quality public chemistry resource for supporting improved predictive toxicology. | |
Molecular Weight |
175.01 g/mol | |
Source | PubChem | |
URL | https://pubchem.ncbi.nlm.nih.gov | |
Description | Data deposited in or computed by PubChem | |
Product Name |
2,3-Dichlorobenzaldehyde | |
CAS RN |
6334-18-5, 31155-09-6 | |
Record name | 2,3-Dichlorobenzaldehyde | |
Source | CAS Common Chemistry | |
URL | https://commonchemistry.cas.org/detail?cas_rn=6334-18-5 | |
Description | CAS Common Chemistry is an open community resource for accessing chemical information. Nearly 500,000 chemical substances from CAS REGISTRY cover areas of community interest, including common and frequently regulated chemicals, and those relevant to high school and undergraduate chemistry classes. This chemical information, curated by our expert scientists, is provided in alignment with our mission as a division of the American Chemical Society. | |
Explanation | The data from CAS Common Chemistry is provided under a CC-BY-NC 4.0 license, unless otherwise stated. | |
Record name | 2,3-Dichlorobenzaldehyde | |
Source | ChemIDplus | |
URL | https://pubchem.ncbi.nlm.nih.gov/substance/?source=chemidplus&sourceid=0006334185 | |
Description | ChemIDplus is a free, web search system that provides access to the structure and nomenclature authority files used for the identification of chemical substances cited in National Library of Medicine (NLM) databases, including the TOXNET system. | |
Record name | Benzaldehyde, dichloro- | |
Source | ChemIDplus | |
URL | https://pubchem.ncbi.nlm.nih.gov/substance/?source=chemidplus&sourceid=0031155096 | |
Description | ChemIDplus is a free, web search system that provides access to the structure and nomenclature authority files used for the identification of chemical substances cited in National Library of Medicine (NLM) databases, including the TOXNET system. | |
Record name | 2,3-Dichlorobenzaldehyde | |
Source | DTP/NCI | |
URL | https://dtp.cancer.gov/dtpstandard/servlet/dwindex?searchtype=NSC&outputformat=html&searchlist=28450 | |
Description | The NCI Development Therapeutics Program (DTP) provides services and resources to the academic and private-sector research communities worldwide to facilitate the discovery and development of new cancer therapeutic agents. | |
Explanation | Unless otherwise indicated, all text within NCI products is free of copyright and may be reused without our permission. Credit the National Cancer Institute as the source. | |
Record name | Benzaldehyde, dichloro- | |
Source | EPA Chemicals under the TSCA | |
URL | https://www.epa.gov/chemicals-under-tsca | |
Description | EPA Chemicals under the Toxic Substances Control Act (TSCA) collection contains information on chemicals and their regulations under TSCA, including non-confidential content from the TSCA Chemical Substance Inventory and Chemical Data Reporting. | |
Record name | Benzaldehyde, 2,3-dichloro- | |
Source | EPA Chemicals under the TSCA | |
URL | https://www.epa.gov/chemicals-under-tsca | |
Description | EPA Chemicals under the Toxic Substances Control Act (TSCA) collection contains information on chemicals and their regulations under TSCA, including non-confidential content from the TSCA Chemical Substance Inventory and Chemical Data Reporting. | |
Record name | 2,3-Dichlorobenzaldehyde | |
Source | EPA DSSTox | |
URL | https://comptox.epa.gov/dashboard/DTXSID7064239 | |
Description | DSSTox provides a high quality public chemistry resource for supporting improved predictive toxicology. | |
Record name | 2,3-dichlorobenzaldehyde | |
Source | European Chemicals Agency (ECHA) | |
URL | https://echa.europa.eu/substance-information/-/substanceinfo/100.026.101 | |
Description | The European Chemicals Agency (ECHA) is an agency of the European Union which is the driving force among regulatory authorities in implementing the EU's groundbreaking chemicals legislation for the benefit of human health and the environment as well as for innovation and competitiveness. | |
Explanation | Use of the information, documents and data from the ECHA website is subject to the terms and conditions of this Legal Notice, and subject to other binding limitations provided for under applicable law, the information, documents and data made available on the ECHA website may be reproduced, distributed and/or used, totally or in part, for non-commercial purposes provided that ECHA is acknowledged as the source: "Source: European Chemicals Agency, http://echa.europa.eu/". Such acknowledgement must be included in each copy of the material. ECHA permits and encourages organisations and individuals to create links to the ECHA website under the following cumulative conditions: Links can only be made to webpages that provide a link to the Legal Notice page. | |
Record name | Dichlorobenzaldehyde | |
Source | European Chemicals Agency (ECHA) | |
URL | https://echa.europa.eu/substance-information/-/substanceinfo/100.045.888 | |
Description | The European Chemicals Agency (ECHA) is an agency of the European Union which is the driving force among regulatory authorities in implementing the EU's groundbreaking chemicals legislation for the benefit of human health and the environment as well as for innovation and competitiveness. | |
Explanation | Use of the information, documents and data from the ECHA website is subject to the terms and conditions of this Legal Notice, and subject to other binding limitations provided for under applicable law, the information, documents and data made available on the ECHA website may be reproduced, distributed and/or used, totally or in part, for non-commercial purposes provided that ECHA is acknowledged as the source: "Source: European Chemicals Agency, http://echa.europa.eu/". Such acknowledgement must be included in each copy of the material. ECHA permits and encourages organisations and individuals to create links to the ECHA website under the following cumulative conditions: Links can only be made to webpages that provide a link to the Legal Notice page. | |
Record name | 2,3-DICHLOROBENZALDEHYDE | |
Source | FDA Global Substance Registration System (GSRS) | |
URL | https://gsrs.ncats.nih.gov/ginas/app/beta/substances/H6GN7Q4PCS | |
Description | The FDA Global Substance Registration System (GSRS) enables the efficient and accurate exchange of information on what substances are in regulated products. Instead of relying on names, which vary across regulatory domains, countries, and regions, the GSRS knowledge base makes it possible for substances to be defined by standardized, scientific descriptions. | |
Explanation | Unless otherwise noted, the contents of the FDA website (www.fda.gov), both text and graphics, are not copyrighted. They are in the public domain and may be republished, reprinted and otherwise used freely by anyone without the need to obtain permission from FDA. Credit to the U.S. Food and Drug Administration as the source is appreciated but not required. | |
Synthesis routes and methods
Procedure details
Retrosynthesis Analysis
AI-Powered Synthesis Planning: Our tool employs the Template_relevance Pistachio, Template_relevance Bkms_metabolic, Template_relevance Pistachio_ringbreaker, Template_relevance Reaxys, Template_relevance Reaxys_biocatalysis model, leveraging a vast database of chemical reactions to predict feasible synthetic routes.
One-Step Synthesis Focus: Specifically designed for one-step synthesis, it provides concise and direct routes for your target compounds, streamlining the synthesis process.
Accurate Predictions: Utilizing the extensive PISTACHIO, BKMS_METABOLIC, PISTACHIO_RINGBREAKER, REAXYS, REAXYS_BIOCATALYSIS database, our tool offers high-accuracy predictions, reflecting the latest in chemical research and data.
Strategy Settings
Precursor scoring | Relevance Heuristic |
---|---|
Min. plausibility | 0.01 |
Model | Template_relevance |
Template Set | Pistachio/Bkms_metabolic/Pistachio_ringbreaker/Reaxys/Reaxys_biocatalysis |
Top-N result to add to graph | 6 |
Feasible Synthetic Routes
Disclaimer and Information on In-Vitro Research Products
Please be aware that all articles and product information presented on BenchChem are intended solely for informational purposes. The products available for purchase on BenchChem are specifically designed for in-vitro studies, which are conducted outside of living organisms. In-vitro studies, derived from the Latin term "in glass," involve experiments performed in controlled laboratory settings using cells or tissues. It is important to note that these products are not categorized as medicines or drugs, and they have not received approval from the FDA for the prevention, treatment, or cure of any medical condition, ailment, or disease. We must emphasize that any form of bodily introduction of these products into humans or animals is strictly prohibited by law. It is essential to adhere to these guidelines to ensure compliance with legal and ethical standards in research and experimentation.