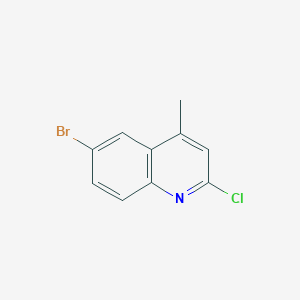
6-Bromo-2-chloro-4-methylquinoline
Overview
Description
6-Bromo-2-chloro-4-methylquinoline is a heterocyclic aromatic compound with the molecular formula C10H7BrClN and a molecular weight of 256.53 g/mol It is a derivative of quinoline, which is a bicyclic compound consisting of a benzene ring fused to a pyridine ring
Preparation Methods
Synthetic Routes and Reaction Conditions: 6-Bromo-2-chloro-4-methylquinoline can be synthesized through various methods. One common method involves the reaction of 6-bromo-4-methylquinolin-2-one with phosphorus oxychloride. The reaction mixture is refluxed for a specific period, followed by cooling and neutralization with sodium bicarbonate . Another method involves the use of microwave irradiation to accelerate the reaction, which significantly reduces the reaction time .
Industrial Production Methods: Industrial production methods for this compound typically involve large-scale synthesis using similar reaction conditions as described above. The use of continuous flow reactors and automated systems can enhance the efficiency and yield of the production process.
Chemical Reactions Analysis
Types of Reactions: 6-Bromo-2-chloro-4-methylquinoline undergoes various chemical reactions, including:
Nucleophilic Substitution: The bromine or chlorine atoms can be substituted by nucleophiles under appropriate conditions.
Oxidation and Reduction: The compound can undergo oxidation and reduction reactions, although specific conditions and reagents are required.
Coupling Reactions: It can participate in coupling reactions such as Suzuki-Miyaura coupling, where it reacts with boronic acids in the presence of a palladium catalyst.
Common Reagents and Conditions:
Nucleophilic Substitution: Common reagents include sodium azide, potassium cyanide, and amines.
Oxidation: Reagents such as potassium permanganate or chromium trioxide can be used.
Reduction: Reducing agents like lithium aluminum hydride or sodium borohydride are commonly employed.
Coupling Reactions: Palladium catalysts and boronic acids are used in Suzuki-Miyaura coupling reactions.
Major Products Formed: The major products formed from these reactions depend on the specific reagents and conditions used. For example, nucleophilic substitution can yield various substituted quinolines, while coupling reactions can produce biaryl compounds.
Scientific Research Applications
6-Bromo-2-chloro-4-methylquinoline has several scientific research applications:
Medicinal Chemistry: It is used as a building block for the synthesis of pharmaceutical compounds with potential antimicrobial, anticancer, and anti-inflammatory properties.
Materials Science: The compound is used in the development of organic semiconductors and light-emitting diodes (LEDs).
Chemical Biology: It serves as a probe for studying biological processes and interactions at the molecular level.
Industrial Applications: It is used in the synthesis of dyes, pigments, and other specialty chemicals.
Mechanism of Action
The mechanism of action of 6-Bromo-2-chloro-4-methylquinoline depends on its specific application. In medicinal chemistry, it may act by inhibiting enzymes or receptors involved in disease pathways. For example, quinoline derivatives are known to inhibit DNA synthesis by promoting the cleavage of bacterial DNA gyrase and type IV topoisomerase, leading to bacterial cell death . The compound may also interact with other molecular targets, such as kinases and ion channels, to exert its effects.
Comparison with Similar Compounds
- 6-Bromo-4-chloro-2-methylquinoline
- 4-Bromo-6-chloro-2-methylquinoline
- 7-Bromo-4-chloro-6-methylquinoline
- 8-Bromo-4-chloro-2-methylquinoline
- 6-Bromo-4-chloro-7-methylquinoline
- 6-Bromo-4-chloro-8-methylquinoline
- 4-Chloro-6-methylquinoline
- 6-Bromo-2-methylquinoline
- 6-Chloro-2-methylquinoline
Comparison: 6-Bromo-2-chloro-4-methylquinoline is unique due to its specific substitution pattern, which can influence its reactivity and properties. Compared to other similar compounds, it may exhibit different biological activities and chemical reactivity, making it valuable for specific applications in research and industry.
Properties
IUPAC Name |
6-bromo-2-chloro-4-methylquinoline | |
---|---|---|
Source | PubChem | |
URL | https://pubchem.ncbi.nlm.nih.gov | |
Description | Data deposited in or computed by PubChem | |
InChI |
InChI=1S/C10H7BrClN/c1-6-4-10(12)13-9-3-2-7(11)5-8(6)9/h2-5H,1H3 | |
Source | PubChem | |
URL | https://pubchem.ncbi.nlm.nih.gov | |
Description | Data deposited in or computed by PubChem | |
InChI Key |
NPDAQPXGOAYKAV-UHFFFAOYSA-N | |
Source | PubChem | |
URL | https://pubchem.ncbi.nlm.nih.gov | |
Description | Data deposited in or computed by PubChem | |
Canonical SMILES |
CC1=CC(=NC2=C1C=C(C=C2)Br)Cl | |
Source | PubChem | |
URL | https://pubchem.ncbi.nlm.nih.gov | |
Description | Data deposited in or computed by PubChem | |
Molecular Formula |
C10H7BrClN | |
Source | PubChem | |
URL | https://pubchem.ncbi.nlm.nih.gov | |
Description | Data deposited in or computed by PubChem | |
DSSTOX Substance ID |
DTXSID80406182 | |
Record name | 6-bromo-2-chloro-4-methylquinoline | |
Source | EPA DSSTox | |
URL | https://comptox.epa.gov/dashboard/DTXSID80406182 | |
Description | DSSTox provides a high quality public chemistry resource for supporting improved predictive toxicology. | |
Molecular Weight |
256.52 g/mol | |
Source | PubChem | |
URL | https://pubchem.ncbi.nlm.nih.gov | |
Description | Data deposited in or computed by PubChem | |
CAS No. |
3913-19-7 | |
Record name | 6-bromo-2-chloro-4-methylquinoline | |
Source | EPA DSSTox | |
URL | https://comptox.epa.gov/dashboard/DTXSID80406182 | |
Description | DSSTox provides a high quality public chemistry resource for supporting improved predictive toxicology. | |
Record name | 6-BROMO-2-CHLORO-4-METHYLQUINOLINE | |
Source | European Chemicals Agency (ECHA) | |
URL | https://echa.europa.eu/information-on-chemicals | |
Description | The European Chemicals Agency (ECHA) is an agency of the European Union which is the driving force among regulatory authorities in implementing the EU's groundbreaking chemicals legislation for the benefit of human health and the environment as well as for innovation and competitiveness. | |
Explanation | Use of the information, documents and data from the ECHA website is subject to the terms and conditions of this Legal Notice, and subject to other binding limitations provided for under applicable law, the information, documents and data made available on the ECHA website may be reproduced, distributed and/or used, totally or in part, for non-commercial purposes provided that ECHA is acknowledged as the source: "Source: European Chemicals Agency, http://echa.europa.eu/". Such acknowledgement must be included in each copy of the material. ECHA permits and encourages organisations and individuals to create links to the ECHA website under the following cumulative conditions: Links can only be made to webpages that provide a link to the Legal Notice page. | |
Synthesis routes and methods I
Procedure details
Synthesis routes and methods II
Procedure details
Retrosynthesis Analysis
AI-Powered Synthesis Planning: Our tool employs the Template_relevance Pistachio, Template_relevance Bkms_metabolic, Template_relevance Pistachio_ringbreaker, Template_relevance Reaxys, Template_relevance Reaxys_biocatalysis model, leveraging a vast database of chemical reactions to predict feasible synthetic routes.
One-Step Synthesis Focus: Specifically designed for one-step synthesis, it provides concise and direct routes for your target compounds, streamlining the synthesis process.
Accurate Predictions: Utilizing the extensive PISTACHIO, BKMS_METABOLIC, PISTACHIO_RINGBREAKER, REAXYS, REAXYS_BIOCATALYSIS database, our tool offers high-accuracy predictions, reflecting the latest in chemical research and data.
Strategy Settings
Precursor scoring | Relevance Heuristic |
---|---|
Min. plausibility | 0.01 |
Model | Template_relevance |
Template Set | Pistachio/Bkms_metabolic/Pistachio_ringbreaker/Reaxys/Reaxys_biocatalysis |
Top-N result to add to graph | 6 |
Feasible Synthetic Routes
Q1: What were the key findings regarding the Knorr synthesis of 6-Bromo-4-methylquinolin-2(1H)-one in the study?
A1: The research rectified some previously unclear aspects of the Knorr synthesis for this specific compound. [] Firstly, the researchers optimized the initial condensation reaction between 4-bromoaniline and β-keto esters by using 1H NMR monitoring. This optimization favored the desired anilide product and minimized the formation of the unwanted crotonate byproduct. [] Secondly, the study uncovered an unanticipated steric effect during the cyclization of anilides to 6-bromoquinolin-2(1H)-one. [] This finding is significant for understanding the reactivity and selectivity of the Knorr reaction in the context of similar compounds. Ultimately, this deeper understanding led to a more efficient three-step synthesis of the final 6-Bromo-2-chloro-4-methylquinoline, achieving a 48% overall yield from 4-bromoaniline. []
Disclaimer and Information on In-Vitro Research Products
Please be aware that all articles and product information presented on BenchChem are intended solely for informational purposes. The products available for purchase on BenchChem are specifically designed for in-vitro studies, which are conducted outside of living organisms. In-vitro studies, derived from the Latin term "in glass," involve experiments performed in controlled laboratory settings using cells or tissues. It is important to note that these products are not categorized as medicines or drugs, and they have not received approval from the FDA for the prevention, treatment, or cure of any medical condition, ailment, or disease. We must emphasize that any form of bodily introduction of these products into humans or animals is strictly prohibited by law. It is essential to adhere to these guidelines to ensure compliance with legal and ethical standards in research and experimentation.