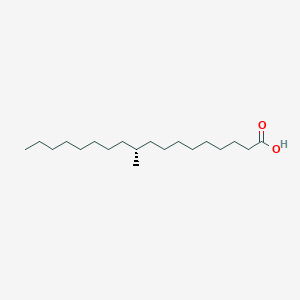
L-(+)-Tuberculostearic acid
- Click on QUICK INQUIRY to receive a quote from our team of experts.
- With the quality product at a COMPETITIVE price, you can focus more on your research.
Overview
Description
(10R)-10-Methyloctadecanoic acid is a unique fatty acid characterized by the presence of a methyl group at the 10th carbon of the octadecanoic acid chain. This compound is notable for its chiral center at the 10th carbon, which gives it specific stereochemical properties. Fatty acids like (10R)-10-methyloctadecanoic acid are essential components of various biological systems and have significant industrial applications.
Preparation Methods
Synthetic Routes and Reaction Conditions: The synthesis of (10R)-10-methyloctadecanoic acid typically involves the selective introduction of a methyl group at the 10th carbon of the octadecanoic acid chain. This can be achieved through various organic synthesis techniques, including:
Grignard Reaction: A Grignard reagent, such as methylmagnesium bromide, can be used to introduce the methyl group at the desired position.
Hydroboration-Oxidation: This method involves the hydroboration of an unsaturated precursor followed by oxidation to introduce the methyl group.
Industrial Production Methods: Industrial production of (10R)-10-methyloctadecanoic acid may involve biotechnological approaches, such as the use of recombinant microorganisms engineered to produce the compound from renewable resources. This method is advantageous due to its sustainability and potential for large-scale production.
Types of Reactions:
Oxidation: (10R)-10-Methyloctadecanoic acid can undergo oxidation reactions to form various oxidized derivatives.
Reduction: Reduction reactions can convert the acid to its corresponding alcohol.
Substitution: The methyl group at the 10th carbon can participate in substitution reactions, leading to the formation of different derivatives.
Common Reagents and Conditions:
Oxidizing Agents: Potassium permanganate (KMnO4) or chromium trioxide (CrO3) can be used for oxidation reactions.
Reducing Agents: Lithium aluminum hydride (LiAlH4) or sodium borohydride (NaBH4) are common reducing agents.
Substitution Reagents: Halogenating agents like thionyl chloride (SOCl2) can be used for substitution reactions.
Major Products:
Oxidation Products: Oxidized derivatives such as ketones or aldehydes.
Reduction Products: Alcohols corresponding to the original acid.
Substitution Products: Halogenated derivatives or other substituted compounds.
Scientific Research Applications
(10R)-10-Methyloctadecanoic acid has a wide range of applications in scientific research:
Chemistry: It is used as a model compound to study stereochemistry and reaction mechanisms.
Biology: The compound is studied for its role in cellular membranes and metabolic pathways.
Medicine: Research is ongoing to explore its potential therapeutic effects and its role in lipid metabolism.
Industry: It is used in the production of specialty chemicals, lubricants, and surfactants.
Mechanism of Action
The mechanism by which (10R)-10-methyloctadecanoic acid exerts its effects involves its incorporation into lipid bilayers and interaction with specific enzymes involved in fatty acid metabolism. The chiral center at the 10th carbon plays a crucial role in its biological activity, influencing its interaction with molecular targets and pathways.
Comparison with Similar Compounds
Stearic Acid: A saturated fatty acid with no methyl substitution.
Oleic Acid: An unsaturated fatty acid with a double bond at the 9th carbon.
Palmitic Acid: A saturated fatty acid with a shorter chain length.
Uniqueness: (10R)-10-Methyloctadecanoic acid is unique due to its chiral center and specific methyl substitution, which confer distinct stereochemical properties and biological activities compared to other fatty acids.
Biological Activity
L-(+)-Tuberculostearic acid (TSA), also known as 10-methyloctadecanoic acid, is a branched-chain fatty acid predominantly found in the membranes of mycobacteria, particularly Mycobacterium tuberculosis (Mtb). Its unique structural properties and biological activities have made it a focal point in tuberculosis research, especially concerning its role as a biomarker for infection and its implications in the pathophysiology of tuberculosis.
1. Structural Characteristics
TSA is characterized by its branched-chain structure, which distinguishes it from other fatty acids. Its molecular formula is C19H38O2, and it plays a crucial role in the structural integrity and functionality of mycobacterial membranes.
2.1. Diagnostic Marker for Tuberculosis
Recent studies have highlighted TSA-containing phosphatidylinositols (PIs) as reliable markers for determining the bacterial burden of Mtb in infected individuals. For instance, a study demonstrated that elevated levels of TSA-containing PIs were detectable in peripheral blood mononuclear cells (PBMCs) from tuberculosis patients compared to healthy controls, indicating its potential use as a rapid diagnostic tool for monitoring TB therapy .
2.2. Role in Mycobacterial Membrane Integrity
TSA is essential for maintaining the structural integrity of mycobacterial membranes. It has been shown to influence membrane partitioning and fluidity, which are critical for mycobacterial survival and pathogenicity. Research indicates that TSA contributes to the lateral organization of membrane domains, which is vital for various cellular processes, including nutrient uptake and response to environmental stressors .
3.1. Interaction with Host Immune Response
TSA has been implicated in modulating host immune responses during Mtb infection. The presence of TSA may influence the activation and function of immune cells, such as macrophages, potentially affecting the outcome of the infection. Studies suggest that mycobacterial lipids, including TSA, can manipulate host signaling pathways, leading to altered inflammatory responses .
3.2. Biosynthesis Pathway
The biosynthesis of TSA involves specific enzymatic pathways within mycobacteria. The enzyme Cfa has been identified as a key methyltransferase responsible for converting oleic acid into TSA. This process is crucial for the production of major membrane phospholipids containing TSA, thus underscoring its importance in mycobacterial lipid metabolism .
4.1. Clinical Relevance
A recent clinical study observed that levels of PI 16:0_19:0 (containing TSA) decreased significantly in TB patients undergoing treatment, correlating with successful therapy outcomes . This finding underscores the potential of TSA as a biomarker not only for diagnosis but also for monitoring treatment efficacy.
4.2. Experimental Models
In experimental models using Mtb-infected mice, researchers noted that TSA levels correlated with bacterial counts in lung tissues, further validating its role as a marker for infection severity .
5. Conclusion
This compound serves as a significant biomarker for tuberculosis due to its unique presence in mycobacterial membranes and its role in modulating host immune responses. Ongoing research continues to explore its potential applications in diagnostics and treatment monitoring, highlighting its importance in understanding and combating tuberculosis.
Properties
CAS No. |
133398-13-7 |
---|---|
Molecular Formula |
C19H38O2 |
Molecular Weight |
298.5 g/mol |
IUPAC Name |
(10R)-10-methyloctadecanoic acid |
InChI |
InChI=1S/C19H38O2/c1-3-4-5-6-9-12-15-18(2)16-13-10-7-8-11-14-17-19(20)21/h18H,3-17H2,1-2H3,(H,20,21)/t18-/m1/s1 |
InChI Key |
BEOUGZFCUMNGOU-GOSISDBHSA-N |
Isomeric SMILES |
CCCCCCCC[C@@H](C)CCCCCCCCC(=O)O |
Canonical SMILES |
CCCCCCCCC(C)CCCCCCCCC(=O)O |
Origin of Product |
United States |
Disclaimer and Information on In-Vitro Research Products
Please be aware that all articles and product information presented on BenchChem are intended solely for informational purposes. The products available for purchase on BenchChem are specifically designed for in-vitro studies, which are conducted outside of living organisms. In-vitro studies, derived from the Latin term "in glass," involve experiments performed in controlled laboratory settings using cells or tissues. It is important to note that these products are not categorized as medicines or drugs, and they have not received approval from the FDA for the prevention, treatment, or cure of any medical condition, ailment, or disease. We must emphasize that any form of bodily introduction of these products into humans or animals is strictly prohibited by law. It is essential to adhere to these guidelines to ensure compliance with legal and ethical standards in research and experimentation.