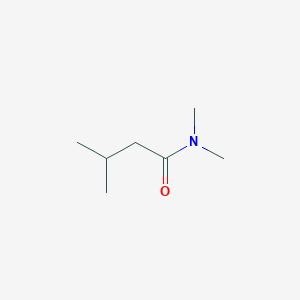
N,N,3-trimethylbutanamide
Overview
Description
N,N,3-Trimethylbutanamide is an organic compound with the molecular formula C₇H₁₅NO. It is known for its unique structure and properties, making it a valuable compound in various scientific and industrial applications. This compound is typically a colorless to slightly yellow transparent liquid with a distinctive odor .
Preparation Methods
Synthetic Routes and Reaction Conditions
N,N,3-Trimethylbutanamide is commonly synthesized through the reaction of N,N-dimethylacetamide with trimethyl ethyl ketone in the presence of a catalyst such as a base. The reaction may involve steps like condensation, hydrolysis, and hydrogenation to yield the final product .
Industrial Production Methods
In industrial settings, the production of this compound follows similar synthetic routes but on a larger scale. The process involves precise control of reaction conditions, including temperature, pressure, and the use of high-purity reagents to ensure the quality and yield of the product .
Chemical Reactions Analysis
Types of Reactions
N,N,3-Trimethylbutanamide undergoes various chemical reactions, including:
Oxidation: This compound can be oxidized under specific conditions to form corresponding oxidized products.
Reduction: It can be reduced to form different amide derivatives.
Substitution: This compound can participate in substitution reactions where functional groups are replaced by other groups.
Common Reagents and Conditions
Oxidation: Common oxidizing agents include potassium permanganate and chromium trioxide.
Reduction: Reducing agents such as lithium aluminum hydride or sodium borohydride are used.
Substitution: Various halogenating agents and nucleophiles are employed in substitution reactions.
Major Products Formed
The major products formed from these reactions depend on the specific reagents and conditions used. For example, oxidation may yield carboxylic acids, while reduction can produce primary or secondary amines .
Scientific Research Applications
N,N,3-Trimethylbutanamide has a wide range of applications in scientific research:
Mechanism of Action
The mechanism of action of N,N,3-trimethylbutanamide involves its interaction with specific molecular targets and pathways. For instance, it may act on enzymes or receptors, modulating their activity and leading to various biochemical effects. The exact pathways and targets depend on the specific application and context of use .
Comparison with Similar Compounds
Similar Compounds
N,N-Dimethylacetamide: A precursor in the synthesis of N,N,3-trimethylbutanamide.
Trimethyl ethyl ketone: Another related compound used in the synthesis process.
Uniqueness
This compound stands out due to its unique structure, which imparts specific chemical and physical properties. Its versatility in various reactions and applications makes it a valuable compound in both research and industry .
Properties
IUPAC Name |
N,N,3-trimethylbutanamide | |
---|---|---|
Source | PubChem | |
URL | https://pubchem.ncbi.nlm.nih.gov | |
Description | Data deposited in or computed by PubChem | |
InChI |
InChI=1S/C7H15NO/c1-6(2)5-7(9)8(3)4/h6H,5H2,1-4H3 | |
Source | PubChem | |
URL | https://pubchem.ncbi.nlm.nih.gov | |
Description | Data deposited in or computed by PubChem | |
InChI Key |
LVQDLURAEDSMES-UHFFFAOYSA-N | |
Source | PubChem | |
URL | https://pubchem.ncbi.nlm.nih.gov | |
Description | Data deposited in or computed by PubChem | |
Canonical SMILES |
CC(C)CC(=O)N(C)C | |
Source | PubChem | |
URL | https://pubchem.ncbi.nlm.nih.gov | |
Description | Data deposited in or computed by PubChem | |
Molecular Formula |
C7H15NO | |
Source | PubChem | |
URL | https://pubchem.ncbi.nlm.nih.gov | |
Description | Data deposited in or computed by PubChem | |
DSSTOX Substance ID |
DTXSID40405050 | |
Record name | N,N,3-trimethylbutanamide | |
Source | EPA DSSTox | |
URL | https://comptox.epa.gov/dashboard/DTXSID40405050 | |
Description | DSSTox provides a high quality public chemistry resource for supporting improved predictive toxicology. | |
Molecular Weight |
129.20 g/mol | |
Source | PubChem | |
URL | https://pubchem.ncbi.nlm.nih.gov | |
Description | Data deposited in or computed by PubChem | |
CAS No. |
5370-28-5 | |
Record name | N,N,3-Trimethylbutanamide | |
Source | CAS Common Chemistry | |
URL | https://commonchemistry.cas.org/detail?cas_rn=5370-28-5 | |
Description | CAS Common Chemistry is an open community resource for accessing chemical information. Nearly 500,000 chemical substances from CAS REGISTRY cover areas of community interest, including common and frequently regulated chemicals, and those relevant to high school and undergraduate chemistry classes. This chemical information, curated by our expert scientists, is provided in alignment with our mission as a division of the American Chemical Society. | |
Explanation | The data from CAS Common Chemistry is provided under a CC-BY-NC 4.0 license, unless otherwise stated. | |
Record name | N,N,3-trimethylbutanamide | |
Source | EPA DSSTox | |
URL | https://comptox.epa.gov/dashboard/DTXSID40405050 | |
Description | DSSTox provides a high quality public chemistry resource for supporting improved predictive toxicology. | |
Retrosynthesis Analysis
AI-Powered Synthesis Planning: Our tool employs the Template_relevance Pistachio, Template_relevance Bkms_metabolic, Template_relevance Pistachio_ringbreaker, Template_relevance Reaxys, Template_relevance Reaxys_biocatalysis model, leveraging a vast database of chemical reactions to predict feasible synthetic routes.
One-Step Synthesis Focus: Specifically designed for one-step synthesis, it provides concise and direct routes for your target compounds, streamlining the synthesis process.
Accurate Predictions: Utilizing the extensive PISTACHIO, BKMS_METABOLIC, PISTACHIO_RINGBREAKER, REAXYS, REAXYS_BIOCATALYSIS database, our tool offers high-accuracy predictions, reflecting the latest in chemical research and data.
Strategy Settings
Precursor scoring | Relevance Heuristic |
---|---|
Min. plausibility | 0.01 |
Model | Template_relevance |
Template Set | Pistachio/Bkms_metabolic/Pistachio_ringbreaker/Reaxys/Reaxys_biocatalysis |
Top-N result to add to graph | 6 |
Feasible Synthetic Routes
Disclaimer and Information on In-Vitro Research Products
Please be aware that all articles and product information presented on BenchChem are intended solely for informational purposes. The products available for purchase on BenchChem are specifically designed for in-vitro studies, which are conducted outside of living organisms. In-vitro studies, derived from the Latin term "in glass," involve experiments performed in controlled laboratory settings using cells or tissues. It is important to note that these products are not categorized as medicines or drugs, and they have not received approval from the FDA for the prevention, treatment, or cure of any medical condition, ailment, or disease. We must emphasize that any form of bodily introduction of these products into humans or animals is strictly prohibited by law. It is essential to adhere to these guidelines to ensure compliance with legal and ethical standards in research and experimentation.