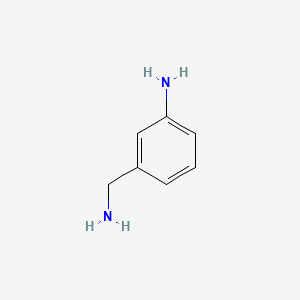
3-Aminobenzylamine
Overview
Description
3-Aminobenzylamine, also known as 3-(aminomethyl)aniline, is an organic compound with the molecular formula C7H10N2. It is a derivative of benzylamine where an amino group is substituted at the meta position of the benzene ring. This compound is a low melting solid or liquid that appears white to light brown in color. It is commonly used in the synthesis of various organic compounds and has applications in multiple fields, including chemistry, biology, and industry .
Mechanism of Action
Biochemical Pathways
3-Aminobenzylamine has been used in the fabrication of an electrochemical dopamine biosensor . In this application, the compound interacts with dopamine, a key neurotransmitter, indicating its potential involvement in biochemical pathways related to neurotransmission .
Result of Action
In the context of its use in a dopamine biosensor, the compound likely interacts with dopamine to produce a measurable signal .
Action Environment
The action of this compound can be influenced by various environmental factors. For instance, the compound is stable under normal temperature and pressure .
Biochemical Analysis
Biochemical Properties
3-Aminobenzylamine plays a significant role in biochemical reactions, particularly in the synthesis of polymers and as an intermediate in organic synthesis. It interacts with enzymes such as monoamine oxidase, which catalyzes the oxidative deamination of amines. This interaction is crucial for the metabolism of neurotransmitters. Additionally, this compound can form Schiff bases with aldehydes and ketones, which are important in various biochemical processes .
Cellular Effects
This compound has been shown to influence various cellular processes. It affects cell signaling pathways by interacting with receptors and enzymes involved in neurotransmitter metabolism. This compound can modulate gene expression by influencing transcription factors and other regulatory proteins. In cellular metabolism, this compound can alter the levels of key metabolites, impacting energy production and biosynthetic pathways .
Molecular Mechanism
The molecular mechanism of this compound involves its interaction with biomolecules through hydrogen bonding, hydrophobic interactions, and covalent bonding. It can inhibit or activate enzymes by binding to their active sites or allosteric sites. For example, this compound can inhibit monoamine oxidase, leading to increased levels of neurotransmitters such as serotonin and dopamine. This inhibition can result in changes in gene expression and cellular responses .
Temporal Effects in Laboratory Settings
In laboratory settings, the effects of this compound can change over time due to its stability and degradation. The compound is relatively stable under standard laboratory conditions, but it can degrade when exposed to light or high temperatures. Long-term studies have shown that this compound can have sustained effects on cellular function, including prolonged enzyme inhibition and changes in gene expression .
Dosage Effects in Animal Models
The effects of this compound vary with different dosages in animal models. At low doses, it can enhance neurotransmitter levels and improve cognitive function. At high doses, it can cause toxic effects, including oxidative stress and cellular damage. Threshold effects have been observed, where a certain dosage is required to achieve a significant biological response .
Metabolic Pathways
This compound is involved in several metabolic pathways, including the catabolism of amines and the synthesis of polymers. It interacts with enzymes such as monoamine oxidase and aldehyde dehydrogenase, which are involved in the breakdown of amines. These interactions can affect metabolic flux and the levels of various metabolites, influencing overall cellular metabolism .
Transport and Distribution
Within cells and tissues, this compound is transported and distributed through various mechanisms. It can be transported across cell membranes by specific transporters or through passive diffusion. Once inside the cell, it can bind to proteins and other biomolecules, affecting its localization and accumulation. The distribution of this compound can influence its biological activity and interactions with other cellular components .
Subcellular Localization
The subcellular localization of this compound is crucial for its activity and function. It can be localized to specific compartments such as the cytoplasm, mitochondria, or nucleus, depending on the presence of targeting signals or post-translational modifications. This localization can affect its interactions with enzymes and other biomolecules, influencing its overall biological effects .
Preparation Methods
Synthetic Routes and Reaction Conditions
3-Aminobenzylamine can be synthesized through several methods:
Reduction of 3-Nitrobenzylamine: One common method involves the reduction of 3-nitrobenzylamine using hydrogen gas in the presence of a catalyst such as palladium on carbon. The reaction is typically carried out under mild conditions, resulting in the formation of this compound.
Amination of Benzyl Halides: Another method involves the amination of benzyl halides with ammonia or primary amines. This reaction can be catalyzed by transition metals such as nickel or palladium.
Hydrogenation of 3-Cyanobenzylamine: 3-Cyanobenzylamine can be hydrogenated in the presence of a catalyst to yield this compound
Industrial Production Methods
Industrial production of this compound often involves the reduction of 3-nitrobenzylamine due to its efficiency and cost-effectiveness. The process is typically carried out in large reactors under controlled conditions to ensure high yield and purity of the product .
Chemical Reactions Analysis
Types of Reactions
3-Aminobenzylamine undergoes various chemical reactions, including:
Oxidation: It can be oxidized to form corresponding imines or nitriles.
Reduction: It can be further reduced to form more substituted amines.
Substitution: It can undergo nucleophilic substitution reactions, where the amino group can be replaced by other functional groups.
Polymerization: It can be polymerized to form poly(this compound), which has applications in biosensors.
Common Reagents and Conditions
Oxidation: Common oxidizing agents include potassium permanganate and hydrogen peroxide.
Reduction: Hydrogen gas in the presence of palladium on carbon is commonly used.
Substitution: Reagents such as alkyl halides and acyl chlorides are used under basic conditions
Major Products Formed
Oxidation: Imines, nitriles.
Reduction: Substituted amines.
Substitution: Various substituted benzylamines
Scientific Research Applications
3-Aminobenzylamine has a wide range of applications in scientific research:
Chemistry: It is used as a building block in the synthesis of various organic compounds, including dyes and pharmaceuticals.
Biology: It is used in the preparation of biosensors for the detection of neurotransmitters such as dopamine.
Industry: It is used in the production of polymers and resins, which have applications in coatings and adhesives
Comparison with Similar Compounds
Similar Compounds
2-Aminobenzylamine: Similar structure but with the amino group at the ortho position.
4-Aminobenzylamine: Similar structure but with the amino group at the para position.
Benzylamine: Lacks the additional amino group on the benzene ring.
Uniqueness
3-Aminobenzylamine is unique due to the position of the amino group on the benzene ring, which affects its reactivity and the types of reactions it can undergo. This positional difference can lead to variations in the physical and chemical properties of the compound, making it suitable for specific applications that other isomers may not be able to fulfill .
Properties
IUPAC Name |
3-(aminomethyl)aniline | |
---|---|---|
Source | PubChem | |
URL | https://pubchem.ncbi.nlm.nih.gov | |
Description | Data deposited in or computed by PubChem | |
InChI |
InChI=1S/C7H10N2/c8-5-6-2-1-3-7(9)4-6/h1-4H,5,8-9H2 | |
Source | PubChem | |
URL | https://pubchem.ncbi.nlm.nih.gov | |
Description | Data deposited in or computed by PubChem | |
InChI Key |
ZDBWYUOUYNQZBM-UHFFFAOYSA-N | |
Source | PubChem | |
URL | https://pubchem.ncbi.nlm.nih.gov | |
Description | Data deposited in or computed by PubChem | |
Canonical SMILES |
C1=CC(=CC(=C1)N)CN | |
Source | PubChem | |
URL | https://pubchem.ncbi.nlm.nih.gov | |
Description | Data deposited in or computed by PubChem | |
Molecular Formula |
C7H10N2 | |
Source | PubChem | |
URL | https://pubchem.ncbi.nlm.nih.gov | |
Description | Data deposited in or computed by PubChem | |
DSSTOX Substance ID |
DTXSID60405032 | |
Record name | 3-Aminobenzylamine | |
Source | EPA DSSTox | |
URL | https://comptox.epa.gov/dashboard/DTXSID60405032 | |
Description | DSSTox provides a high quality public chemistry resource for supporting improved predictive toxicology. | |
Molecular Weight |
122.17 g/mol | |
Source | PubChem | |
URL | https://pubchem.ncbi.nlm.nih.gov | |
Description | Data deposited in or computed by PubChem | |
CAS No. |
4403-70-7 | |
Record name | 3-Aminobenzylamine | |
Source | CAS Common Chemistry | |
URL | https://commonchemistry.cas.org/detail?cas_rn=4403-70-7 | |
Description | CAS Common Chemistry is an open community resource for accessing chemical information. Nearly 500,000 chemical substances from CAS REGISTRY cover areas of community interest, including common and frequently regulated chemicals, and those relevant to high school and undergraduate chemistry classes. This chemical information, curated by our expert scientists, is provided in alignment with our mission as a division of the American Chemical Society. | |
Explanation | The data from CAS Common Chemistry is provided under a CC-BY-NC 4.0 license, unless otherwise stated. | |
Record name | 3-Aminobenzylamine | |
Source | ChemIDplus | |
URL | https://pubchem.ncbi.nlm.nih.gov/substance/?source=chemidplus&sourceid=0004403707 | |
Description | ChemIDplus is a free, web search system that provides access to the structure and nomenclature authority files used for the identification of chemical substances cited in National Library of Medicine (NLM) databases, including the TOXNET system. | |
Record name | 3-Aminobenzylamine | |
Source | EPA DSSTox | |
URL | https://comptox.epa.gov/dashboard/DTXSID60405032 | |
Description | DSSTox provides a high quality public chemistry resource for supporting improved predictive toxicology. | |
Record name | 3-aminobenzylamine | |
Source | European Chemicals Agency (ECHA) | |
URL | https://echa.europa.eu/substance-information/-/substanceinfo/100.101.159 | |
Description | The European Chemicals Agency (ECHA) is an agency of the European Union which is the driving force among regulatory authorities in implementing the EU's groundbreaking chemicals legislation for the benefit of human health and the environment as well as for innovation and competitiveness. | |
Explanation | Use of the information, documents and data from the ECHA website is subject to the terms and conditions of this Legal Notice, and subject to other binding limitations provided for under applicable law, the information, documents and data made available on the ECHA website may be reproduced, distributed and/or used, totally or in part, for non-commercial purposes provided that ECHA is acknowledged as the source: "Source: European Chemicals Agency, http://echa.europa.eu/". Such acknowledgement must be included in each copy of the material. ECHA permits and encourages organisations and individuals to create links to the ECHA website under the following cumulative conditions: Links can only be made to webpages that provide a link to the Legal Notice page. | |
Record name | Benzenemethanamine, 3-amino | |
Source | European Chemicals Agency (ECHA) | |
URL | https://echa.europa.eu/substance-information/-/substanceinfo/100.130.142 | |
Description | The European Chemicals Agency (ECHA) is an agency of the European Union which is the driving force among regulatory authorities in implementing the EU's groundbreaking chemicals legislation for the benefit of human health and the environment as well as for innovation and competitiveness. | |
Explanation | Use of the information, documents and data from the ECHA website is subject to the terms and conditions of this Legal Notice, and subject to other binding limitations provided for under applicable law, the information, documents and data made available on the ECHA website may be reproduced, distributed and/or used, totally or in part, for non-commercial purposes provided that ECHA is acknowledged as the source: "Source: European Chemicals Agency, http://echa.europa.eu/". Such acknowledgement must be included in each copy of the material. ECHA permits and encourages organisations and individuals to create links to the ECHA website under the following cumulative conditions: Links can only be made to webpages that provide a link to the Legal Notice page. | |
Synthesis routes and methods I
Procedure details
Synthesis routes and methods II
Procedure details
Synthesis routes and methods III
Procedure details
Synthesis routes and methods IV
Procedure details
Synthesis routes and methods V
Procedure details
Retrosynthesis Analysis
AI-Powered Synthesis Planning: Our tool employs the Template_relevance Pistachio, Template_relevance Bkms_metabolic, Template_relevance Pistachio_ringbreaker, Template_relevance Reaxys, Template_relevance Reaxys_biocatalysis model, leveraging a vast database of chemical reactions to predict feasible synthetic routes.
One-Step Synthesis Focus: Specifically designed for one-step synthesis, it provides concise and direct routes for your target compounds, streamlining the synthesis process.
Accurate Predictions: Utilizing the extensive PISTACHIO, BKMS_METABOLIC, PISTACHIO_RINGBREAKER, REAXYS, REAXYS_BIOCATALYSIS database, our tool offers high-accuracy predictions, reflecting the latest in chemical research and data.
Strategy Settings
Precursor scoring | Relevance Heuristic |
---|---|
Min. plausibility | 0.01 |
Model | Template_relevance |
Template Set | Pistachio/Bkms_metabolic/Pistachio_ringbreaker/Reaxys/Reaxys_biocatalysis |
Top-N result to add to graph | 6 |
Feasible Synthetic Routes
Disclaimer and Information on In-Vitro Research Products
Please be aware that all articles and product information presented on BenchChem are intended solely for informational purposes. The products available for purchase on BenchChem are specifically designed for in-vitro studies, which are conducted outside of living organisms. In-vitro studies, derived from the Latin term "in glass," involve experiments performed in controlled laboratory settings using cells or tissues. It is important to note that these products are not categorized as medicines or drugs, and they have not received approval from the FDA for the prevention, treatment, or cure of any medical condition, ailment, or disease. We must emphasize that any form of bodily introduction of these products into humans or animals is strictly prohibited by law. It is essential to adhere to these guidelines to ensure compliance with legal and ethical standards in research and experimentation.