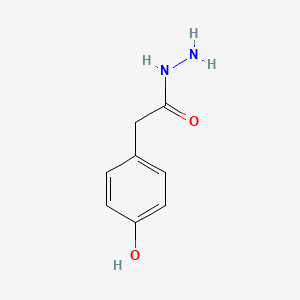
2-(4-Hydroxyphenyl)acetohydrazide
Overview
Description
2-(4-Hydroxyphenyl)acetohydrazide is an organic compound with the molecular formula C8H10N2O2. It is a derivative of acetohydrazide, where the acetyl group is substituted with a 4-hydroxyphenyl group. This compound is known for its applications in various fields, including chemistry, biology, and medicine.
Preparation Methods
Synthetic Routes and Reaction Conditions
2-(4-Hydroxyphenyl)acetohydrazide can be synthesized through several methods. One common synthetic route involves the reaction of 4-hydroxybenzaldehyde with hydrazine hydrate to form 4-hydroxybenzaldehyde hydrazone. This intermediate is then reacted with acetic anhydride to yield this compound. The reaction conditions typically involve refluxing the reactants in an appropriate solvent such as ethanol or methanol.
Industrial Production Methods
In industrial settings, the production of this compound may involve similar synthetic routes but on a larger scale. The use of continuous flow reactors and optimized reaction conditions can enhance the yield and purity of the final product. Additionally, purification techniques such as recrystallization or chromatography are employed to obtain high-purity this compound.
Chemical Reactions Analysis
Types of Reactions
2-(4-Hydroxyphenyl)acetohydrazide undergoes various chemical reactions, including:
Oxidation: The hydroxyl group can be oxidized to form a ketone or aldehyde derivative.
Reduction: The carbonyl group can be reduced to form an alcohol derivative.
Substitution: The hydrazide group can participate in nucleophilic substitution reactions, leading to the formation of various derivatives.
Common Reagents and Conditions
Oxidation: Common oxidizing agents include potassium permanganate and chromium trioxide.
Reduction: Reducing agents such as sodium borohydride or lithium aluminum hydride are used.
Substitution: Nucleophiles such as amines or thiols can be used under mild conditions to achieve substitution reactions.
Major Products Formed
Oxidation: 4-Hydroxybenzaldehyde or 4-hydroxyacetophenone derivatives.
Reduction: 2-(4-Hydroxyphenyl)ethanol derivatives.
Substitution: Various substituted hydrazides depending on the nucleophile used.
Scientific Research Applications
2-(4-Hydroxyphenyl)acetohydrazide has several scientific research applications:
Chemistry: Used as a building block in the synthesis of more complex organic molecules.
Biology: Investigated for its potential as an enzyme inhibitor or as a ligand in biochemical assays.
Medicine: Explored for its potential therapeutic properties, including anti-inflammatory and anticancer activities.
Industry: Utilized in the development of new materials and as a precursor in the synthesis of dyes and pigments.
Mechanism of Action
The mechanism of action of 2-(4-Hydroxyphenyl)acetohydrazide involves its interaction with specific molecular targets. For instance, it may inhibit certain enzymes by binding to their active sites, thereby blocking their catalytic activity. Additionally, it can interact with cellular receptors, modulating signaling pathways and exerting biological effects. The exact molecular targets and pathways depend on the specific application and context of use.
Comparison with Similar Compounds
2-(4-Hydroxyphenyl)acetohydrazide can be compared with other similar compounds, such as:
2-(4-Methoxyphenyl)acetohydrazide: Similar structure but with a methoxy group instead of a hydroxyl group. This compound may exhibit different reactivity and biological activity.
2-(4-Chlorophenyl)acetohydrazide:
2-(4-Nitrophenyl)acetohydrazide: The presence of a nitro group can significantly alter the compound’s reactivity and biological effects.
The uniqueness of this compound lies in its hydroxyl group, which imparts specific chemical reactivity and potential biological activity that may not be present in its analogs.
Properties
IUPAC Name |
2-(4-hydroxyphenyl)acetohydrazide | |
---|---|---|
Source | PubChem | |
URL | https://pubchem.ncbi.nlm.nih.gov | |
Description | Data deposited in or computed by PubChem | |
InChI |
InChI=1S/C8H10N2O2/c9-10-8(12)5-6-1-3-7(11)4-2-6/h1-4,11H,5,9H2,(H,10,12) | |
Source | PubChem | |
URL | https://pubchem.ncbi.nlm.nih.gov | |
Description | Data deposited in or computed by PubChem | |
InChI Key |
YJKVPKGOZNHONU-UHFFFAOYSA-N | |
Source | PubChem | |
URL | https://pubchem.ncbi.nlm.nih.gov | |
Description | Data deposited in or computed by PubChem | |
Canonical SMILES |
C1=CC(=CC=C1CC(=O)NN)O | |
Source | PubChem | |
URL | https://pubchem.ncbi.nlm.nih.gov | |
Description | Data deposited in or computed by PubChem | |
Molecular Formula |
C8H10N2O2 | |
Source | PubChem | |
URL | https://pubchem.ncbi.nlm.nih.gov | |
Description | Data deposited in or computed by PubChem | |
DSSTOX Substance ID |
DTXSID30395658 | |
Record name | 2-(4-hydroxyphenyl)acetohydrazide | |
Source | EPA DSSTox | |
URL | https://comptox.epa.gov/dashboard/DTXSID30395658 | |
Description | DSSTox provides a high quality public chemistry resource for supporting improved predictive toxicology. | |
Molecular Weight |
166.18 g/mol | |
Source | PubChem | |
URL | https://pubchem.ncbi.nlm.nih.gov | |
Description | Data deposited in or computed by PubChem | |
CAS No. |
20277-02-5 | |
Record name | 2-(4-hydroxyphenyl)acetohydrazide | |
Source | EPA DSSTox | |
URL | https://comptox.epa.gov/dashboard/DTXSID30395658 | |
Description | DSSTox provides a high quality public chemistry resource for supporting improved predictive toxicology. | |
Synthesis routes and methods I
Procedure details
Synthesis routes and methods II
Procedure details
Synthesis routes and methods III
Procedure details
Retrosynthesis Analysis
AI-Powered Synthesis Planning: Our tool employs the Template_relevance Pistachio, Template_relevance Bkms_metabolic, Template_relevance Pistachio_ringbreaker, Template_relevance Reaxys, Template_relevance Reaxys_biocatalysis model, leveraging a vast database of chemical reactions to predict feasible synthetic routes.
One-Step Synthesis Focus: Specifically designed for one-step synthesis, it provides concise and direct routes for your target compounds, streamlining the synthesis process.
Accurate Predictions: Utilizing the extensive PISTACHIO, BKMS_METABOLIC, PISTACHIO_RINGBREAKER, REAXYS, REAXYS_BIOCATALYSIS database, our tool offers high-accuracy predictions, reflecting the latest in chemical research and data.
Strategy Settings
Precursor scoring | Relevance Heuristic |
---|---|
Min. plausibility | 0.01 |
Model | Template_relevance |
Template Set | Pistachio/Bkms_metabolic/Pistachio_ringbreaker/Reaxys/Reaxys_biocatalysis |
Top-N result to add to graph | 6 |
Feasible Synthetic Routes
Q1: How does the structure of 2-(4-Hydroxyphenyl)acetohydrazide influence its ability to form complexes with metal ions?
A1: this compound possesses several features conducive to metal complexation. The molecule contains both a hydrazide group (-C(O)NHNH2) and a phenolic hydroxyl group (-OH) on the phenyl ring. [, ] These functional groups can act as electron donors, readily forming coordinate bonds with metal ions. Research has shown that this compound can chelate metal ions like Cadmium (Cd2+), creating stable complexes. [] The specific coordination geometry and stability of these complexes are influenced by factors like the metal ion's size, charge, and preferred coordination number, as well as the presence of other ligands in the system.
Q2: What insights do the reported studies provide about the potential applications of this compound and its derivatives?
A2: The studies highlight the versatility of this compound and its derivatives for various applications. For instance, the fluorescence properties of the Cd(II) complex with a ligand derived from this compound, as described in the first paper, suggest potential applications in areas like fluorescent probes and sensors. [] Furthermore, understanding the thermal stability of these complexes, as investigated through thermogravimetric analysis, is crucial for applications involving heat treatments or high-temperature environments. [] While the second paper focuses on the interaction of similar acyl hydrazones with calf thymus DNA (CT-DNA), it underscores the potential of these compounds for developing novel DNA-binding agents. [] These findings contribute valuable insights into the structure-activity relationship, paving the way for designing more potent and selective compounds for various biomedical applications.
Disclaimer and Information on In-Vitro Research Products
Please be aware that all articles and product information presented on BenchChem are intended solely for informational purposes. The products available for purchase on BenchChem are specifically designed for in-vitro studies, which are conducted outside of living organisms. In-vitro studies, derived from the Latin term "in glass," involve experiments performed in controlled laboratory settings using cells or tissues. It is important to note that these products are not categorized as medicines or drugs, and they have not received approval from the FDA for the prevention, treatment, or cure of any medical condition, ailment, or disease. We must emphasize that any form of bodily introduction of these products into humans or animals is strictly prohibited by law. It is essential to adhere to these guidelines to ensure compliance with legal and ethical standards in research and experimentation.