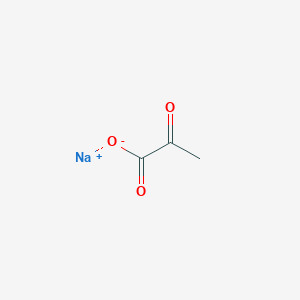
Sodium pyruvate
Overview
Description
Chemical Structure and Properties
Sodium pyruvate (C₃H₃NaO₃) is the sodium salt of pyruvic acid, a three-carbon α-keto acid critical in glycolysis and the tricarboxylic acid (TCA) cycle. It has a molecular weight of 110.04 g/mol and is highly soluble in water (up to 100 mM) but less so in organic solvents . Its structure includes a ketone group at the α-carbon, enabling its role as a metabolic intermediate and antioxidant .
Applications
this compound is widely used in:
Preparation Methods
Synthetic Routes and Reaction Conditions: One method for preparing sodium pyruvate involves recrystallization. The process includes dissolving a crude product of this compound in purified water, filtering to remove inorganic impurities, and then crystallizing and precipitating this compound by adding the solution to an ice bath containing anhydrous ethanol . The wet product is then dried under reduced pressure, sieved, and pulverized to obtain the pure white solid this compound .
Industrial Production Methods: Industrial production of this compound often involves the chemical synthesis of pyruvic acid, which is then neutralized with sodium hydroxide to form this compound. This process can be optimized for high yield and purity, making it suitable for large-scale production .
Chemical Reactions Analysis
Types of Reactions: Sodium pyruvate undergoes various chemical reactions, including:
Oxidation: this compound can be oxidized to produce acetate, water, and carbon dioxide.
Reduction: It can be reduced to form lactate under anaerobic conditions.
Substitution: this compound can participate in substitution reactions, where the pyruvate anion acts as a nucleophile.
Common Reagents and Conditions:
Oxidation: Hydrogen peroxide is a common reagent used in the oxidation of this compound.
Reduction: Lactate dehydrogenase is an enzyme that catalyzes the reduction of pyruvate to lactate.
Major Products:
Oxidation: Acetate, water, and carbon dioxide.
Reduction: Lactate.
Scientific Research Applications
Cell Culture Applications
Sodium pyruvate is commonly used in cell culture media as an energy source. It enhances cell viability and growth by supplying additional pyruvate, which is crucial for cellular respiration and metabolism.
Case Study: In a study involving bovine oocytes, this compound significantly promoted nuclear maturation when used in non-serum maturation media, highlighting its importance in reproductive biology .
Medical and Clinical Applications
This compound has been investigated for its potential benefits in various medical scenarios, particularly in resuscitation and mitochondrial diseases.
Resuscitation Fluid
Recent studies have shown that this compound may be superior to traditional resuscitation fluids like sodium chloride. In a rat model of hemorrhagic shock, 90% of animals treated with this compound survived compared to only 30% receiving sodium chloride .
Mitochondrial Disease Treatment
This compound has been explored as a therapeutic agent for patients with mitochondrial disorders. A study monitored four bedridden patients receiving this compound (0.5-1.0 g/kg/day) for over 12 months, revealing that three patients showed significant improvements within 1-3 months of treatment . This suggests that this compound may help restore glycolytic function by decreasing the NADH/NAD(+) ratio.
Neuroprotection
This compound exhibits neuroprotective properties, particularly in models of hypoxic-ischemic injury. Research indicates that it can reduce injury to neonatal rat brains under hypoxic conditions, suggesting its potential for treating neonatal brain injuries .
Antioxidant Properties
The compound's ability to scavenge free radicals enhances its application in various biological systems. In studies involving Staphylococcus aureus, this compound improved cytotoxicity under high glucose conditions by mitigating oxidative stress .
Fibrosis and Metabolic Disorders
Recent findings suggest that this compound may improve mitochondrial fitness in fibroblasts from systemic sclerosis (SSc) patients. It inhibits the transition from fibroblasts to myofibroblasts, which is crucial in fibrotic remodeling processes .
Enhancing Drug Delivery Systems
This compound's properties have been utilized to enhance drug delivery systems in cancer treatment. For instance, it has been combined with nanoparticles to improve the efficacy of chemotherapy agents against glioblastoma cells .
Mechanism of Action
Sodium pyruvate exerts its effects through several mechanisms:
Energy Production: It is converted to acetyl coenzyme A, which enters the tricarboxylic acid (TCA) cycle to produce ATP.
Antioxidant Properties: this compound scavenges reactive oxygen species (ROS) such as hydrogen peroxide, reducing oxidative stress.
Metabolic Pathways: It plays a role in glycolysis and gluconeogenesis, influencing glucose metabolism and energy production.
Comparison with Similar Compounds
Comparison with Similar Compounds
Ethyl Pyruvate (EP)
Key Findings :
- SP outperforms EP in correcting lactic acidosis due to its immediate alkalizing action .
- EP exhibits stronger anti-inflammatory effects in sepsis, likely due to ester-mediated cellular uptake .
Other α-Keto Acid Derivatives
- Oxobutyrate and Oxovalerate: Structural Difference: Longer aliphatic chains compared to pyruvate. Binding Affinity: Higher affinity for pyruvate-binding proteins (e.g., TakP) due to increased hydrophobicity . Applications: Potential drug carriers for targeted delivery .
Acetylphosphinate :
Lactate
Key Findings :
Sodium Bicarbonate
Key Findings :
Data Tables
Table 1: Kinetic Parameters of LDH for Pyruvate in Diabetic vs. Healthy Models
Model | Km (Pyruvate) | Vmax (nmol/min/10⁶ RBCs) | Reference |
---|---|---|---|
Healthy RBCs | 0.16 mM | 12.5 ± 1.2 | |
Type II Diabetic RBCs | 0.32 mM | 8.7 ± 0.9 |
Table 2: Cytotoxicity of S. aureus in Different Media
Medium | This compound (10 mM) | Cytotoxicity (Erythrocyte Lysis, %) | Reference |
---|---|---|---|
LB (Aerobic) | Yes | 85 ± 6 | |
LB (Anaerobic) | Yes | 12 ± 3 |
Research Findings and Mechanisms
- Neuroprotection : SP and EP reduce hippocampal neuronal loss post-TBI by enhancing cytochrome oxidase activity and suppressing microglial activation .
- Metabolic Effects : SP activates HIF-1α-EPO signaling, improving oxygen utilization in hypoxia .
- Enzyme Interactions : Pyruvate derivatives with longer carbon chains (e.g., oxovalerate) bind more effectively to transport proteins, suggesting design principles for drug analogs .
Biological Activity
Sodium pyruvate, the sodium salt of pyruvic acid, is a compound with significant biological activity, particularly in the fields of metabolism, neuroprotection, and cellular stress response. This article explores the diverse biological effects of this compound, supported by various studies and case reports.
- Antioxidant Properties : this compound exhibits potent antioxidant effects, particularly in protecting mitochondria from oxidative stress. Studies have shown that it significantly reduces cell death in neuroblastoma cells exposed to hydrogen peroxide (H₂O₂) by decreasing reactive oxygen species (ROS) generation and stabilizing mitochondrial membrane potential .
- Metabolic Effects : this compound plays a crucial role in cellular metabolism by acting as a substrate for energy production. It enhances the NAD⁺/NADH ratio, which is vital for various metabolic processes including glycolysis and the tricarboxylic acid (TCA) cycle .
- Neuroprotection : Research indicates that this compound can protect neuronal cells from damage due to oxidative stress and ischemia. In vivo studies have demonstrated its ability to improve survival rates and cerebral energetic parameters in models of hemorrhagic shock .
Table 1: Summary of Key Studies on this compound
Neuroprotective Effects
A pivotal study demonstrated that this compound co-treatment significantly increased cell survival in neuroblastoma SK-N-SH cells subjected to oxidative stress. The protective effect was dose-dependent, with concentrations as low as 1 mM providing substantial protection against H₂O₂-induced cytotoxicity . Furthermore, delayed administration of this compound up to 2 hours post-insult still conferred protective benefits, indicating its potential therapeutic window.
Metabolic Resuscitation
This compound's role in metabolic resuscitation has been highlighted in several clinical settings. For instance, a case report noted its effectiveness in managing severe lactic acidosis in a child with Leigh syndrome through high-dose oral administration . This aligns with findings that this compound can enhance ATP levels and prolong cellular survival during anoxic conditions.
Potential Clinical Applications
The unique properties of this compound suggest several potential clinical applications:
- Critical Care : this compound-enriched fluids may improve outcomes in critically ill patients by correcting metabolic acidosis and enhancing cellular energy metabolism.
- Neuroprotection : Its ability to protect against oxidative stress opens avenues for research into treatments for neurodegenerative diseases.
- Cancer Therapy : The modulation of histone gene expression by this compound may provide a novel approach to cancer treatment by inhibiting tumor growth.
Q & A
Basic Research Questions
Q. What is the role of sodium pyruvate in cell culture media, and how should its concentration be optimized?
this compound serves as a critical energy substrate in cell culture, supporting glycolysis and the tricarboxylic acid (TCA) cycle. It provides a carbon source for acetyl-CoA synthesis, essential for ATP production under aerobic conditions . In anaerobic environments, pyruvate is reduced to lactate, aiding NAD+ regeneration. For mesenchymal stem cell cultures, a 1 mM concentration (110 mg/mL) is standard, as used in MEM medium with 10% FBS . Optimization involves titration experiments (e.g., 0.5–2 mM) to balance metabolic demand and avoid pH shifts from lactate accumulation.
Q. How can researchers verify the purity and stability of this compound in experimental preparations?
The USP-compliant assay involves iodometric titration: dissolving this compound in water, reacting with 0.05 M iodine under alkaline conditions, and titrating excess iodine with sodium thiosulfate. Purity ≥97% is confirmed when 1 mL of 0.05 M iodine corresponds to 1.834 mg C₃H₃NaO₃ . Stability testing requires monitoring pH (5.0–7.0), osmolarity (300–500 mOsm/kg), and sterility over 18 months at 2–8°C .
Q. What are the methodological considerations for using this compound in oxidative stress studies?
this compound acts as an antioxidant by scavenging reactive oxygen species (ROS) via direct neutralization and indirect upregulation of glutathione synthesis. In vitro, pre-treatment with 5–10 mM pyruvate for 24 hours before inducing oxidative stress (e.g., H₂O₂ exposure) is common. Post-treatment efficacy depends on ROS kinetics, with real-time assays (e.g., DCFH-DA for intracellular ROS) recommended to validate timing .
Advanced Research Questions
Q. How does this compound improve outcomes in traumatic brain injury (TBI) models, and what are key experimental design considerations?
- Dose timing : Delayed administration (e.g., day 4 post-injury) aligns with secondary metabolic dysfunction phases.
Q. What mechanisms underlie conflicting results in pyruvate’s hepatoprotective effects across studies?
Discrepancies arise from route-specific toxicity and co-administered agents. Oral pyruvate (6.6 mg/100 g bw) with dihydroxyacetone reduces ethanol-induced liver fat in rats, but intravenous doses (80 mM via jugular vein) caused mortality due to high alkalinity (56 mM NaOH) and unmonitored physiological stress . Methodological reconciliation requires:
- Toxicity screening : Pre-testing formulations for pH and osmolarity.
- Route optimization : Tailoring delivery (oral vs. IV) to target tissue bioavailability.
Q. How can this compound be integrated into hyperpolarized MRI studies, and what are technical challenges?
Hyperpolarized [13C]pyruvate enables real-time metabolic imaging (e.g., monitoring conversion to lactate in tumors). Key challenges include:
- Polarization stability : Rapid decay (<1–2 minutes) necessitates immediate post-injection imaging.
- Dosing : Preclinical studies use 0.3 mL/s injection rates via tail vein, but central venous routes (e.g., carotid artery) risk hemodynamic stress . Solutions include dynamic nuclear polarization (DNP) optimization and physiological monitoring during infusion.
Q. What experimental frameworks address pyruvate’s dual role as a metabolic substrate and epigenetic modulator?
Pyruvate influences histone acetylation via acetyl-CoA availability. To study this, combine:
- Metabolomics : LC-MS quantification of intracellular acetyl-CoA.
- Chromatin assays : ChIP-seq for H3K27ac marks after pyruvate deprivation/replenishment. Example: In cancer models, 10 mM pyruvate increases acetyl-CoA by 40%, altering gene expression in <6 hours . Controls should include acetate (an alternative acetyl source) to isolate pyruvate-specific effects.
Q. Methodological Guidelines
Properties
CAS No. |
113-24-6 |
---|---|
Molecular Formula |
C3H4NaO3 |
Molecular Weight |
111.05 g/mol |
IUPAC Name |
sodium;2-oxopropanoate |
InChI |
InChI=1S/C3H4O3.Na/c1-2(4)3(5)6;/h1H3,(H,5,6); |
InChI Key |
DAQHMCWYXJEOCG-UHFFFAOYSA-N |
SMILES |
CC(=O)C(=O)[O-].[Na+] |
Isomeric SMILES |
CC(=O)C(=O)[O-].[Na+] |
Canonical SMILES |
CC(=O)C(=O)O.[Na] |
Key on ui other cas no. |
113-24-6 |
physical_description |
Liquid; Dry Powder White to pale yellow powder; [Alfa Aesar MSDS] |
Pictograms |
Irritant |
Synonyms |
2-Oxopropanoic Acid Sodium Salt; Sodium Pyruvate; Sodium α-Ketopropionate; |
Origin of Product |
United States |
Retrosynthesis Analysis
AI-Powered Synthesis Planning: Our tool employs the Template_relevance Pistachio, Template_relevance Bkms_metabolic, Template_relevance Pistachio_ringbreaker, Template_relevance Reaxys, Template_relevance Reaxys_biocatalysis model, leveraging a vast database of chemical reactions to predict feasible synthetic routes.
One-Step Synthesis Focus: Specifically designed for one-step synthesis, it provides concise and direct routes for your target compounds, streamlining the synthesis process.
Accurate Predictions: Utilizing the extensive PISTACHIO, BKMS_METABOLIC, PISTACHIO_RINGBREAKER, REAXYS, REAXYS_BIOCATALYSIS database, our tool offers high-accuracy predictions, reflecting the latest in chemical research and data.
Strategy Settings
Precursor scoring | Relevance Heuristic |
---|---|
Min. plausibility | 0.01 |
Model | Template_relevance |
Template Set | Pistachio/Bkms_metabolic/Pistachio_ringbreaker/Reaxys/Reaxys_biocatalysis |
Top-N result to add to graph | 6 |
Feasible Synthetic Routes
Disclaimer and Information on In-Vitro Research Products
Please be aware that all articles and product information presented on BenchChem are intended solely for informational purposes. The products available for purchase on BenchChem are specifically designed for in-vitro studies, which are conducted outside of living organisms. In-vitro studies, derived from the Latin term "in glass," involve experiments performed in controlled laboratory settings using cells or tissues. It is important to note that these products are not categorized as medicines or drugs, and they have not received approval from the FDA for the prevention, treatment, or cure of any medical condition, ailment, or disease. We must emphasize that any form of bodily introduction of these products into humans or animals is strictly prohibited by law. It is essential to adhere to these guidelines to ensure compliance with legal and ethical standards in research and experimentation.