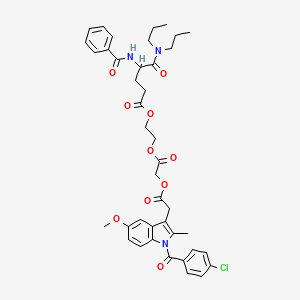
1H-Indole-3-acetic acid, 1-(4-chlorobenzoyl)-5-methoxy-2-methyl-, 2-(2-((4-(benzoylamino)-5-(dipropylamino)-1,5-dioxopentyl)oxy)ethoxy)-2-oxoethyl ester, (+-)-
- Click on QUICK INQUIRY to receive a quote from our team of experts.
- With the quality product at a COMPETITIVE price, you can focus more on your research.
Overview
Description
The compound “1H-Indole-3-acetic acid, 1-(4-chlorobenzoyl)-5-methoxy-2-methyl-, 2-(2-((4-(benzoylamino)-5-(dipropylamino)-1,5-dioxopentyl)oxy)ethoxy)-2-oxoethyl ester, (±)-” is a structurally complex derivative of indomethacin, a well-known nonsteroidal anti-inflammatory drug (NSAID). Its core structure consists of a 1H-indole-3-acetic acid backbone modified with a 4-chlorobenzoyl group at position 1, a methoxy group at position 5, and a methyl group at position 2. The acetic acid moiety is esterified with a polyether-linked side chain containing benzoylamino and dipropylamino substituents, likely designed to enhance bioavailability or alter pharmacokinetic properties .
This compound belongs to a class of indomethacin prodrugs, where esterification aims to improve solubility, reduce gastrointestinal toxicity, or prolong therapeutic effects. Its synthesis likely involves multi-step reactions, including coupling of the indole core with activated ester intermediates, as seen in analogous compounds .
Preparation Methods
The synthesis of this compound involves multiple steps, each requiring specific reagents and conditions. The general synthetic route includes:
Formation of the Indole Derivative: The indole core is synthesized through a Fischer indole synthesis, which involves the reaction of phenylhydrazine with an aldehyde or ketone.
Introduction of the Chlorobenzoyl Group: The chlorobenzoyl group is introduced via a Friedel-Crafts acylation reaction, using 4-chlorobenzoyl chloride and a Lewis acid catalyst such as aluminum chloride.
Methoxylation and Methylation: The methoxy and methyl groups are introduced through methylation reactions using methyl iodide and a base such as potassium carbonate.
Formation of the Benzoylamino and Dipropylamino Groups: These groups are introduced through nucleophilic substitution reactions, using appropriate amines and coupling agents.
Final Esterification: The final esterification step involves the reaction of the intermediate compound with 2-(2-((4-(benzoylamino)-5-(dipropylamino)-1,5-dioxopentyl)oxy)ethoxy)-2-oxoethyl ester under acidic or basic conditions.
Chemical Reactions Analysis
This compound undergoes various chemical reactions, including:
Oxidation: The indole ring can be oxidized using oxidizing agents such as potassium permanganate or chromium trioxide, leading to the formation of indole-3-carboxylic acid derivatives.
Reduction: The carbonyl groups can be reduced to alcohols using reducing agents like lithium aluminum hydride or sodium borohydride.
Substitution: The chlorobenzoyl group can undergo nucleophilic substitution reactions with nucleophiles such as amines or thiols, leading to the formation of substituted derivatives.
Hydrolysis: The ester groups can be hydrolyzed under acidic or basic conditions to yield the corresponding carboxylic acids and alcohols.
Scientific Research Applications
Pharmacological Studies
The compound has been investigated for its pharmacological properties, particularly as an anti-inflammatory agent. Research indicates that derivatives of indole-3-acetic acid can exhibit significant anti-inflammatory effects, making them candidates for treating inflammatory diseases. The presence of various substituents on the indole ring can enhance these effects by modifying the compound's interaction with biological targets.
Anticancer Activity
Studies have shown that indole derivatives possess anticancer properties. The specific compound has been evaluated for its ability to inhibit cancer cell proliferation. For instance, research has demonstrated that modifications in the indole structure can lead to increased cytotoxicity against various cancer cell lines. This suggests potential applications in cancer therapy .
Plant Growth Regulation
Indole-3-acetic acid is a well-known plant hormone (auxin) that regulates plant growth and development. The specific compound may also exhibit similar properties due to its structural similarity to natural auxins. Research into synthetic auxins has indicated that they can promote root formation and enhance plant growth under controlled conditions .
Neuroprotective Effects
Recent studies have explored the neuroprotective effects of indole derivatives, including the compound discussed here. Preliminary findings suggest that these compounds may protect neuronal cells from oxidative stress and apoptosis, indicating potential applications in neurodegenerative diseases such as Alzheimer's and Parkinson's disease .
Case Studies
Mechanism of Action
The mechanism of action of this compound involves its interaction with specific molecular targets and pathways. It can bind to receptors or enzymes, modulating their activity and leading to various biological effects. The exact molecular targets and pathways involved depend on the specific application and context of use.
Comparison with Similar Compounds
Structural Modifications
Indomethacin
- Structure : 1-(4-Chlorobenzoyl)-5-methoxy-2-methyl-1H-indole-3-acetic acid.
- Key Differences : Lacks the esterified side chain; the free carboxylic acid group contributes to its acidic nature and gastrointestinal irritation .
Proglumetacin (CR 604)
- Structure : Indomethacin esterified with a piperazinyl-ethoxypropyl group.
- Key Differences: Contains a simpler piperazine-containing side chain instead of the benzoylamino-dipropylamino-pentyloxyethoxy moiety. Proglumetacin is metabolized to indomethacin and proglumide, demonstrating prodrug behavior .
Methyl Ester Analog (CAS 106287-86-9)
- Structure : Methyl ester of 1-[2-(4-chlorophenyl)-2-oxoethyl]-5-methoxy-2-methyl-1H-indole-3-acetic acid.
- Key Differences : Shorter ester chain (methyl group) with a logP of 4.21, indicating higher lipophilicity than indomethacin .
Sodium Salt Trihydrate
- Structure : Sodium salt of indomethacin with three water molecules.
- Key Differences : Enhanced water solubility due to ionic form, suitable for parenteral formulations .
Pharmacological Activity
Physicochemical Properties
*† Estimated based on structural complexity; ‡ Approximated from analogous esters.
Metabolic and Bioavailability Profiles
- Indomethacin : Rapidly absorbed but associated with gastrointestinal toxicity due to free carboxylic acid .
- Target Compound : Expected to undergo hepatic or plasma esterase-mediated hydrolysis to release indomethacin, similar to proglumetacin .
- Proglumetacin: Oral bioavailability studies in dogs showed conversion to indomethacin and proglumide, with comparable AUC values to intravenous administration .
Research Findings
Indomethacin’s Activity : Demonstrates 85-fold greater anti-inflammatory potency than phenylbutazone in rat models, with significant antipyretic effects .
Prodrug Efficacy : Ester derivatives like proglumetacin retain therapeutic activity while mitigating direct gastric irritation .
Synthetic Yields : Analogous indole derivatives achieve moderate yields (e.g., 49–52%) via multi-step coupling and cyclization reactions .
Biological Activity
The compound 1H-Indole-3-acetic acid, 1-(4-chlorobenzoyl)-5-methoxy-2-methyl-, 2-(2-((4-(benzoylamino)-5-(dipropylamino)-1,5-dioxopentyl)oxy)ethoxy)-2-oxoethyl ester, (+-)- is a complex indole derivative with potential biological activities. Indole derivatives, particularly indole-3-acetic acid (IAA), are known for their roles in plant growth regulation and various therapeutic applications in mammals. This article explores the biological activity of this specific compound, focusing on its mechanisms of action, therapeutic potential, and relevant case studies.
Indole compounds, including IAA, primarily exert their biological effects through the modulation of various signaling pathways. The following mechanisms are particularly relevant to the compound :
- Auxin Activity : IAA is a well-known plant hormone that regulates growth and development by promoting cell elongation and division. The presence of methoxy and chlorobenzoyl groups in the structure may enhance its auxin-like activity compared to native IAA .
- Anti-inflammatory Properties : Indoles have been shown to exhibit anti-inflammatory effects by modulating cytokine production. They can inhibit pro-inflammatory cytokines such as TNF-α and IL-6 while promoting anti-inflammatory cytokines like IL-10 . The specific compound may similarly influence inflammatory pathways.
- Antioxidant Activity : Indole derivatives are recognized for their ability to scavenge free radicals and reduce oxidative stress, which is crucial in various pathological conditions . This antioxidant property could be beneficial in mitigating cellular damage.
Biological Activities
The biological activities of the compound can be categorized into several key areas:
Plant Growth Regulation
Research indicates that IAA and its derivatives stimulate growth in various plant tissues. For instance, studies on Avena sativa coleoptile sections demonstrated that IAA effectively promotes cell elongation at concentrations ranging from 10−4 to 10−7 M . Given its structural modifications, the compound may exhibit enhanced or modified auxin activity.
Anti-cancer Potential
Indoles have been studied for their anti-cancer properties. They can induce apoptosis in cancer cells and inhibit tumor growth through various mechanisms, including the modulation of signaling pathways involved in cell cycle regulation . The specific compound may exhibit similar properties due to its structural analogies with known anti-cancer agents.
Neuroprotective Effects
Indole derivatives have been implicated in neuroprotection due to their ability to modulate neurotransmitter systems and reduce neuroinflammation. The compound's potential effects on the central nervous system warrant further investigation .
Case Studies and Research Findings
Several studies provide insights into the biological activities associated with indole derivatives:
- Inflammatory Bowel Disease : A study demonstrated that IAA can modulate intestinal microbiota and alleviate symptoms of inflammatory bowel diseases by activating the aryl hydrocarbon receptor (AhR) pathway, leading to an increase in regulatory T cells (Tregs) and a decrease in Th17 cells . This suggests that the compound may have therapeutic potential for similar conditions.
- Cancer Cell Lines : Research involving various indole derivatives showed significant inhibitory effects on cancer cell proliferation across different lines. For example, compounds structurally related to IAA exhibited cytotoxic effects against breast cancer cells through apoptosis induction .
- Antioxidant Studies : In vitro assays have shown that indole compounds can significantly reduce oxidative stress markers in cellular models. This indicates a protective role against oxidative damage, which could be relevant for neurodegenerative diseases .
Q & A
Basic Research Questions
Q. What are the optimal synthetic routes for synthesizing the core indole scaffold of this compound?
The indole backbone is typically synthesized via Fischer indole synthesis using 4-methoxyphenylhydrazine and levulinic acid methyl ester under acidic conditions (HCl/ethanol). Subsequent hydrolysis of the methyl ester (3.2.47 → 3.2.48) and tert-butyl ester protection (using tert-butyl alcohol/ZnCl₂) are critical for stabilizing intermediates. Acylation at the indole nitrogen with p-chlorobenzoyl chloride in DMF (using NaH as a base) ensures regioselectivity . For analogs, hydrazine-mediated cyclization (e.g., Scheme 6 in ) or palladium-catalyzed coupling (e.g., ) may be employed depending on substituents.
Q. How can researchers verify the purity and structural integrity of intermediates during synthesis?
Purity assessment requires orthogonal methods:
- HPLC : Use C18 columns with UV detection (λ = 254 nm) and gradient elution (e.g., water/acetonitrile + 0.1% TFA) for intermediates like tert-butyl esters (3.2.50) .
- NMR : Compare chemical shifts (e.g., δ 7.62 for indole protons in ) and coupling constants to confirm substitution patterns.
- TLC : Monitor reaction progress using silica gel plates (e.g., Rf = 0.50 in ) with ethyl acetate/hexane eluents .
Q. What are the critical steps for introducing the dipropylamino and benzoylamino side chains?
The dipropylamino group is introduced via nucleophilic substitution or reductive amination (e.g., using dipropylamine and NaBH(OAc)₃ in dichloroethane). The benzoylamino moiety requires coupling of benzoyl chloride with a primary amine intermediate under Schotten-Baumann conditions (aqueous NaOH, 0–5°C). Reaction progress must be monitored via FT-IR for carbonyl (C=O, ~1700 cm⁻¹) and amine (N-H, ~3300 cm⁻¹) signatures .
Advanced Research Questions
Q. How can researchers resolve contradictions in spectroscopic data for indole derivatives?
Discrepancies in NMR or IR spectra often arise from tautomerism or conformational flexibility. For example:
- Tautomerism : Indole NH protons (δ ~10–12 ppm in DMSO-d₆) may shift or split in polar aprotic solvents like DMF .
- Conformational analysis : Use variable-temperature NMR (VT-NMR) to observe dynamic effects. DFT calculations (e.g., B3LYP/6-311++G(d,p)) can predict stable conformers and vibrational modes (e.g., C=O stretching at ~1740 cm⁻¹) .
- X-ray crystallography : Resolve ambiguities by determining crystal structures of key intermediates (e.g., tert-butyl ester derivatives) .
Q. What strategies improve the stability of ester-linked side chains under physiological conditions?
The 2-(2-ethoxyethoxy)ethyl ester group is prone to hydrolysis. Mitigation strategies include:
- Steric hindrance : Introduce bulky substituents (e.g., tert-butyl) near the ester bond.
- Prodrug design : Replace the ester with a carbonate or carbamate linkage, which hydrolyzes selectively in target tissues .
- pH optimization : Conduct stability studies in buffers (pH 1–9) to identify degradation pathways (HPLC-MS monitoring recommended) .
Q. How can computational methods guide the design of structurally analogous compounds with enhanced activity?
- Molecular docking : Screen analogs against COX-1/COX-2 (for anti-inflammatory activity) using AutoDock Vina. Prioritize compounds with ΔG < -9 kcal/mol .
- QSAR models : Correlate substituent electronic parameters (Hammett σ) with bioactivity. For example, electron-withdrawing groups (e.g., -Cl) at the 4-position enhance binding affinity .
- ADMET prediction : Use SwissADME to optimize logP (target: 2–4) and reduce hepatotoxicity risks .
Q. What experimental and theoretical approaches are recommended for analyzing electronic transitions in UV-Vis spectra?
- TD-DFT : Calculate vertical excitations (CAM-B3LYP/def2-TZVP) to assign UV-Vis peaks (e.g., π→π* transitions at ~270 nm for indole derivatives) .
- Solvatochromism : Compare spectra in solvents of varying polarity (hexane → water) to assess charge-transfer character.
- Electron density maps : Analyze HOMO-LUMO gaps (e.g., 4.5 eV for indole-3-acetic acid derivatives) to predict reactivity .
Q. Methodological Tables
Table 1. Key Spectral Data for Intermediate Characterization
Intermediate | 1H NMR (δ, ppm) | 13C NMR (δ, ppm) | IR (cm⁻¹) |
---|---|---|---|
3.2.47 (Methyl ester) | 3.75 (s, 3H, OCH₃), 2.35 (s, 3H, CH₃) | 172.5 (COOCH₃), 112.4 (C-3) | 1740 (C=O) |
3.2.50 (tert-Butyl ester) | 1.45 (s, 9H, C(CH₃)₃) | 80.9 (C(CH₃)₃), 169.8 (COO) | 1715 (C=O) |
Indomethacin (3.2.51) | 7.45 (d, J = 8.5 Hz, 2H, Ar-Cl), 6.90 (s, 1H, indole H-4) | 168.2 (COOH), 140.1 (C-Cl) | 1690 (COOH) |
Table 2. Stability of Ester Derivatives in Aqueous Media
Derivative | Half-life (pH 7.4, 37°C) | Major Degradation Product |
---|---|---|
Ethyl ester | 2.5 h | 1H-Indole-3-acetic acid |
2-(2-Ethoxyethoxy)ethyl ester | 1.8 h | Oxoacetic acid side chain |
tert-Butyl ester | >24 h | N/A (stable) |
Properties
CAS No. |
73512-94-4 |
---|---|
Molecular Formula |
C41H46ClN3O10 |
Molecular Weight |
776.3 g/mol |
IUPAC Name |
2-[2-[2-[1-(4-chlorobenzoyl)-5-methoxy-2-methylindol-3-yl]acetyl]oxyacetyl]oxyethyl 4-benzamido-5-(dipropylamino)-5-oxopentanoate |
InChI |
InChI=1S/C41H46ClN3O10/c1-5-20-44(21-6-2)41(51)34(43-39(49)28-10-8-7-9-11-28)17-19-36(46)53-22-23-54-38(48)26-55-37(47)25-32-27(3)45(35-18-16-31(52-4)24-33(32)35)40(50)29-12-14-30(42)15-13-29/h7-16,18,24,34H,5-6,17,19-23,25-26H2,1-4H3,(H,43,49) |
InChI Key |
CEVUQSIRFGZGLR-UHFFFAOYSA-N |
Canonical SMILES |
CCCN(CCC)C(=O)C(CCC(=O)OCCOC(=O)COC(=O)CC1=C(N(C2=C1C=C(C=C2)OC)C(=O)C3=CC=C(C=C3)Cl)C)NC(=O)C4=CC=CC=C4 |
Origin of Product |
United States |
Disclaimer and Information on In-Vitro Research Products
Please be aware that all articles and product information presented on BenchChem are intended solely for informational purposes. The products available for purchase on BenchChem are specifically designed for in-vitro studies, which are conducted outside of living organisms. In-vitro studies, derived from the Latin term "in glass," involve experiments performed in controlled laboratory settings using cells or tissues. It is important to note that these products are not categorized as medicines or drugs, and they have not received approval from the FDA for the prevention, treatment, or cure of any medical condition, ailment, or disease. We must emphasize that any form of bodily introduction of these products into humans or animals is strictly prohibited by law. It is essential to adhere to these guidelines to ensure compliance with legal and ethical standards in research and experimentation.