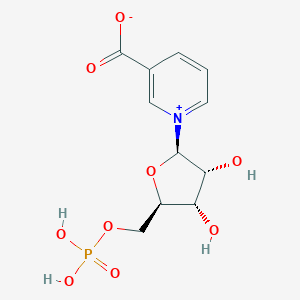
nicotinic acid mononucleotide
Overview
Description
Nicotinic acid D-ribonucleotide is a D-ribonucleotide having nicotinic acid as the nucleobase. It has a role as an Escherichia coli metabolite and a mouse metabolite. It is a conjugate acid of a nicotinate D-ribonucleotide(2-).
Nicotinate mononucleotide is a natural product found in Caenorhabditis elegans with data available.
nicotinic acid D-ribonucleotide is a metabolite found in or produced by Saccharomyces cerevisiae.
Mechanism of Action
Target of Action
NaMN primarily targets the Nicotinate-nucleotide–dimethylbenzimidazole phosphoribosyltransferase in Salmonella typhimurium and Nicotinate-nucleotide pyrophosphorylase [carboxylating] in Mycobacterium tuberculosis . It also targets ADP-ribosyl cyclase 2 , Nicotinamide mononucleotide adenylyltransferase 1 , and Nicotinamide mononucleotide adenylyltransferase 3 in humans .
Mode of Action
NaMN is a key enzyme in all organisms, catalyzing the coupling of ATP and NMN or NaMN, yielding NAD or NaAD, respectively . It plays a central role in NAD+ biosynthesis in all living organisms .
Biochemical Pathways
NaMN is involved in the NAD+ metabolism, a co-enzyme involved in a great deal of biochemical reactions . In mammalian cells, NAD+ is synthesized, predominantly through NMN, to replenish the consumption by NADase participating in physiological processes including DNA repair, metabolism, and cell death . The conversion of dietary nicotinic acid (niacin) and nicotinamide to bioactive NAD+ involves the biosynthesis pathway . Formation of NAD+ from nicotinamide (NAM) occurs in a two-step process and its formation from nicotinic acid occurs in a three-step process .
Pharmacokinetics
It is known that namn is an orally bioavailable nad+ precursor . It is ultimately converted to NAD+, a redox cofactor that mediates many metabolic enzymes .
Result of Action
The administration of NMN has demonstrated amelioration of the pathological conditions in some age-related disease mouse models . The increased amount of NAD+ was strongly correlated with pulse rate before the administration of NMN .
Action Environment
It is known that the nad+ production and consumption pathways, including nmn, are essential for more precise understanding and therapy of age-related pathological processes such as diabetes, ischemia–reperfusion injury, heart failure, alzheimer’s disease, and retinal degeneration .
Biochemical Analysis
Biochemical Properties
Nicotinic acid mononucleotide plays a significant role in biochemical reactions as a precursor to NAD+. It interacts with several enzymes, including nicotinamide phosphoribosyltransferase (NAMPT), which catalyzes the conversion of nicotinamide to this compound. This compound is further converted to NAD+ by nicotinamide mononucleotide adenylyltransferase (NMNAT). These interactions are crucial for maintaining NAD+ levels in cells, which are essential for various metabolic processes .
Cellular Effects
This compound influences various cellular processes by modulating NAD+ levels. It affects cell signaling pathways, gene expression, and cellular metabolism. Increased levels of this compound can enhance NAD+ synthesis, leading to improved mitochondrial function, enhanced DNA repair, and increased resistance to oxidative stress. These effects are particularly significant in aging cells, where NAD+ levels tend to decline .
Molecular Mechanism
At the molecular level, this compound exerts its effects by participating in the NAD+ biosynthesis pathway. It binds to NAMPT, facilitating the conversion of nicotinamide to this compound. This compound is then converted to NAD+ by NMNAT. The increased NAD+ levels activate sirtuins, a family of NAD±dependent deacetylases, which play a crucial role in regulating gene expression, DNA repair, and metabolic processes .
Temporal Effects in Laboratory Settings
In laboratory settings, the effects of this compound have been observed to change over time. The stability and degradation of this compound can influence its long-term effects on cellular function. Studies have shown that this compound is relatively stable under physiological conditions, but its degradation can lead to a decrease in NAD+ levels over time. Long-term studies in vitro and in vivo have demonstrated that sustained supplementation with this compound can maintain NAD+ levels and improve cellular function .
Dosage Effects in Animal Models
The effects of this compound vary with different dosages in animal models. Low to moderate doses have been shown to increase NAD+ levels and improve metabolic functions without adverse effects. High doses can lead to toxic effects, including liver damage and oxidative stress. These findings highlight the importance of determining the optimal dosage for therapeutic applications of this compound .
Metabolic Pathways
This compound is involved in the NAD+ biosynthesis pathway, which includes both de novo and salvage pathways. In the de novo pathway, this compound is synthesized from tryptophan, while in the salvage pathway, it is produced from nicotinamide. The key enzymes involved in these pathways include NAMPT and NMNAT. This compound also interacts with other cofactors, such as ATP, which is required for its conversion to NAD+ .
Transport and Distribution
Within cells, this compound is transported and distributed through specific transporters and binding proteins. The Slc12a8 transporter is known to facilitate the uptake of this compound into cells. Once inside the cell, it is distributed to various cellular compartments, including the cytoplasm and mitochondria, where it participates in NAD+ biosynthesis. The localization and accumulation of this compound are crucial for its function in maintaining NAD+ levels .
Subcellular Localization
This compound is localized in various subcellular compartments, including the cytoplasm and mitochondria. Its activity and function are influenced by its subcellular localization. For instance, in the mitochondria, this compound is involved in maintaining mitochondrial NAD+ levels, which are essential for mitochondrial function and energy production. Targeting signals and post-translational modifications may direct this compound to specific compartments, ensuring its proper function .
Properties
CAS No. |
321-02-8 |
---|---|
Molecular Formula |
C11H15NO9P+ |
Molecular Weight |
336.21 g/mol |
IUPAC Name |
1-[(2R,3R,4S,5R)-3,4-dihydroxy-5-(phosphonooxymethyl)oxolan-2-yl]pyridin-1-ium-3-carboxylic acid |
InChI |
InChI=1S/C11H14NO9P/c13-8-7(5-20-22(17,18)19)21-10(9(8)14)12-3-1-2-6(4-12)11(15)16/h1-4,7-10,13-14H,5H2,(H2-,15,16,17,18,19)/p+1/t7-,8-,9-,10-/m1/s1 |
InChI Key |
JOUIQRNQJGXQDC-ZYUZMQFOSA-O |
SMILES |
C1=CC(=C[N+](=C1)C2C(C(C(O2)COP(=O)(O)[O-])O)O)C(=O)O |
Isomeric SMILES |
C1=CC(=C[N+](=C1)[C@H]2[C@@H]([C@@H]([C@H](O2)COP(=O)(O)O)O)O)C(=O)O |
Canonical SMILES |
C1=CC(=C[N+](=C1)C2C(C(C(O2)COP(=O)(O)O)O)O)C(=O)O |
physical_description |
Solid |
Synonyms |
3-Carboxy-1-(5-O-phosphono-β-D-ribofuranosyl)pyridinium Inner Salt; 3-Carboxy-1-β-D-ribofuranosylpyridinium Hydroxide 5’-Phosphate Inner Salt; Nicotinate Mononucleotide; Nicotinate Ribonucleotide; Nicotinic Acid Ribonucleotide; Nicotinic Acid Riboti |
Origin of Product |
United States |
Retrosynthesis Analysis
AI-Powered Synthesis Planning: Our tool employs the Template_relevance Pistachio, Template_relevance Bkms_metabolic, Template_relevance Pistachio_ringbreaker, Template_relevance Reaxys, Template_relevance Reaxys_biocatalysis model, leveraging a vast database of chemical reactions to predict feasible synthetic routes.
One-Step Synthesis Focus: Specifically designed for one-step synthesis, it provides concise and direct routes for your target compounds, streamlining the synthesis process.
Accurate Predictions: Utilizing the extensive PISTACHIO, BKMS_METABOLIC, PISTACHIO_RINGBREAKER, REAXYS, REAXYS_BIOCATALYSIS database, our tool offers high-accuracy predictions, reflecting the latest in chemical research and data.
Strategy Settings
Precursor scoring | Relevance Heuristic |
---|---|
Min. plausibility | 0.01 |
Model | Template_relevance |
Template Set | Pistachio/Bkms_metabolic/Pistachio_ringbreaker/Reaxys/Reaxys_biocatalysis |
Top-N result to add to graph | 6 |
Feasible Synthetic Routes
Q1: What is the primary function of nicotinic acid mononucleotide (NaMN) in cellular processes?
A1: NaMN is a crucial intermediate in the biosynthesis of nicotinamide adenine dinucleotide (NAD+), an essential cofactor involved in various metabolic reactions and cellular signaling pathways [, , ].
Q2: Can you elaborate on the specific pathways involved in NAD+ biosynthesis where NaMN plays a role?
A2: NaMN participates in both the de novo and salvage pathways of NAD+ synthesis. In the de novo pathway, NaMN is adenylated to nicotinic acid adenine dinucleotide (NaAD), which is subsequently amidated to NAD+ [, , ]. In the salvage pathway, NaMN can be directly converted to NAD+ [].
Q3: Are there any alternative pathways for NAD+ biosynthesis involving NaMN?
A3: Yes, research on Francisella tularensis revealed an alternative pathway where NaMN is first amidated to nicotinamide mononucleotide (NMN) before being adenylated to NAD+ []. This pathway differs from the canonical route observed in Bacillus anthracis, where NaMN is adenylated prior to amidation [].
Q4: How does the administration of nicotinic acid riboside (NAR) and the NMN biosynthesis inhibitor FK866 contribute to axon protection?
A4: This combination promotes an alternative NAD+ generation pathway that utilizes NAMN as an intermediate instead of NMN []. This bypasses NMN formation, effectively protecting axons from degeneration [].
Q5: Does the accumulation of NMN directly cause axon degeneration?
A5: While NMN accumulation has been proposed as a potential cause, research indicates that it is not sufficient by itself to trigger axon degeneration []. Maintaining NAD+ levels alone is also insufficient to protect neurons from degeneration induced by chemotherapeutic agents like vincristine [].
Q6: How does the gut microbiome influence the assimilation of orally delivered NaMN?
A6: Research suggests that the gut microbiome plays a role in deamidating orally administered NMN into NaMN before it is absorbed and incorporated into the host's system []. This process highlights the complex interplay between the host and its gut microbiome in NAD+ metabolism.
Q7: Is the incorporation of exogenous NMN into NAD+ the primary mechanism by which it impacts the NAD+ metabolome?
A7: Evidence suggests that exogenous NMN affects the NAD+ metabolome through indirect mechanisms rather than direct incorporation. One such mechanism is the increased production of endogenous NR in the gut, particularly in microbiome-depleted animals treated with NMN [].
Q8: What is the molecular formula and weight of NaMN?
A8: The molecular formula of NaMN is C11H15N2O8P, and its molecular weight is 346.22 g/mol.
Q9: Can you describe the structural characteristics of NaMN based on its chemical formula?
A9: NaMN comprises a nicotinic acid moiety attached to a ribose sugar, which is further phosphorylated at the 5' position. The presence of carboxyl and phosphate groups contributes to its negative charge at physiological pH.
Q10: How does NaMN interact with enzymes involved in NAD+ biosynthesis?
A10: NaMN interacts with enzymes like this compound adenylyltransferase (NaMNAT) [, , , , , ]. Crystal structures of NaMNAT from various organisms reveal that NaMN binds to the active site, facilitating the transfer of an adenyl group from ATP [, , , ].
Q11: Are there any structural insights into how NaMN interacts with NaMNAT to facilitate catalysis?
A11: Structural studies on Bacillus anthracis NaMNAT (BA NaMNAT) show that NaMN binding occurs within a flexible substrate-binding area surrounded by three loops []. These loops exhibit conformational heterogeneity, potentially influencing substrate binding and catalysis [].
Q12: What is the significance of the conformational heterogeneity observed in the loops surrounding the NaMN binding site of NaMNAT?
A12: This flexibility might be crucial for the enzyme's ability to bind both NaMN and ATP, the two substrates involved in the adenylation reaction. The conformational changes upon substrate binding could contribute to the enzyme's catalytic efficiency.
Q13: Beyond NaMNAT, are there other enzymes with which NaMN interacts?
A13: Yes, NaMN can interact with nicotinamide mononucleotide synthetase, an enzyme found in Francisella tularensis []. This enzyme exhibits an unusual substrate preference for NaMN over NaAD, catalyzing its amidation to NMN [].
Q14: Does NaMN exhibit any inhibitory effects on enzymes?
A14: Interestingly, NaMN acts as a competitive inhibitor of nicotinic acid phosphoribosyltransferase (NAPRTase) mutants []. This inhibition suggests that the NAMN synthesis reaction might occur at a site overlapping with the ATP binding site [].
Q15: What is the structural basis for the opposing effects of NaMN and NMN on SARM1 activity?
A16: Analysis of the NaMN-ARM domain co-crystal structure reveals that NaMN competes with NMN for binding to the SARM1 allosteric site []. This competition suggests that the structural differences between NaMN and NMN dictate their contrasting effects on SARM1 conformation and activity [].
Q16: What are the implications of understanding the structure and function of enzymes involved in NaMN metabolism for drug discovery?
A17: Enzymes like NaMNAT, essential for NAD+ biosynthesis and utilizing NaMN as a substrate, are considered potential targets for developing novel antibiotics [, , ]. Inhibiting these enzymes could disrupt NAD+ production, crucial for bacterial survival, and potentially combat bacterial infections.
Q17: Are there any specific structural features of NaMNAT that make it an attractive target for drug development?
A18: Structural analysis of Mycobacterium tuberculosis NadD (MtNadD) revealed an over-closed conformation maintained by a unique 310 helix []. This conformation renders the enzyme inactive []. Designing inhibitors that lock NaMNAT in this inactive state could lead to potent antibacterial agents.
Q18: Can you describe any experimental techniques used to study NaMN and its role in NAD+ biosynthesis?
A19: Researchers utilize various techniques to investigate NaMN, including: * Isotope labeling studies: Tracking the incorporation of labeled precursors like nicotinic acid into NaMN and subsequently into NAD+ helps elucidate the biosynthetic pathways []. * Enzyme assays: Measuring the activity of enzymes involved in NaMN metabolism, such as NaMNAT and NAPRTase, provides insights into their kinetic parameters and substrate specificity [, , , , , ]. * Crystallography: Determining the three-dimensional structures of enzymes like NaMNAT in complex with substrates or inhibitors provides valuable information about their interactions and potential for drug development [, , , , , ].
Q19: Are there any specific challenges associated with studying NaMN and its associated metabolic pathways?
A20: One challenge is the labile nature of NaMN, as observed in studies on nicotinate mononucleotide:5,6-dimethylbenzimidazole phosphoribosyltransferase (CobT) []. NaMN tends to hydrolyze in the crystal lattice without its co-substrate, making it difficult to obtain stable complexes for structural studies [].
Q20: How do researchers overcome the challenge of NaMN hydrolysis during structural investigations?
A21: One approach is to utilize substrate analogs that mimic NaMN binding but are not susceptible to hydrolysis. For instance, in CobT studies, analogs like 4,5-dimethyl-1,2-phenylenediamine successfully captured the enzyme-substrate complex by excluding water molecules responsible for hydrolysis from the active site [].
Q21: Beyond its role in NAD+ biosynthesis, are there other areas of research where NaMN is being investigated?
A22: NaMN is being explored for its potential in: * Axon protection: Studies show that manipulating NaMN levels, such as through the combined administration of NAR and FK866, can protect neurons from chemotherapy-induced degeneration []. * Metabolic engineering: Engineering enzymes like Escherichia coli NaMN adenylyltransferase to favor NMN over NaMN aims to enhance the amidated pathway activity for NAD+ biosynthesis, offering potential applications in biocatalysis and metabolic engineering [].
Disclaimer and Information on In-Vitro Research Products
Please be aware that all articles and product information presented on BenchChem are intended solely for informational purposes. The products available for purchase on BenchChem are specifically designed for in-vitro studies, which are conducted outside of living organisms. In-vitro studies, derived from the Latin term "in glass," involve experiments performed in controlled laboratory settings using cells or tissues. It is important to note that these products are not categorized as medicines or drugs, and they have not received approval from the FDA for the prevention, treatment, or cure of any medical condition, ailment, or disease. We must emphasize that any form of bodily introduction of these products into humans or animals is strictly prohibited by law. It is essential to adhere to these guidelines to ensure compliance with legal and ethical standards in research and experimentation.