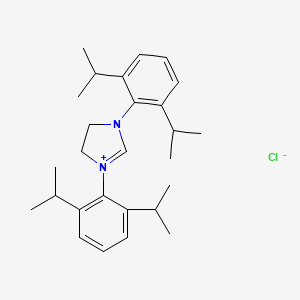
1,3-Bis(2,6-diisopropylphenyl)-4,5-dihydro-1H-imidazol-3-ium chloride
- Click on QUICK INQUIRY to receive a quote from our team of experts.
- With the quality product at a COMPETITIVE price, you can focus more on your research.
Overview
Description
1,3-Bis(2,6-diisopropylphenyl)-4,5-dihydro-1H-imidazol-3-ium chloride is an organic salt known for its unique properties, including excellent thermal stability, good solubility in organic solvents, and notable catalytic activity . This compound is widely used in organic synthesis, especially in the catalytic cycloisomerization of 2-(iodoethynyl)aryl esters .
Mechanism of Action
Target of Action
The primary target of 1,3-Bis(2,6-diisopropylphenyl)-4,5-dihydro-1H-imidazol-3-ium chloride is the palladium acetate . This compound is used with palladium acetate to generate an N-heterocyclic carbene catalyst .
Mode of Action
This compound interacts with its target, palladium acetate, to form a catalyst that can efficiently catalyze the Suzuki-Miyaura coupling of aryl chlorides with aryl boronic acids .
Biochemical Pathways
The compound plays a significant role in the Suzuki-Miyaura coupling reaction, a type of cross-coupling reaction, used in organic synthesis . This reaction is part of the broader class of palladium-catalyzed coupling reactions which form carbon-carbon bonds .
Result of Action
The result of the action of this compound is the formation of an efficient catalyst that can facilitate the Suzuki-Miyaura coupling of aryl chlorides with aryl boronic acids . This leads to the formation of biaryl compounds, which are commonly found in pharmaceuticals and organic materials .
Action Environment
The action of this compound can be influenced by environmental factors. For instance, the compound is hygroscopic , meaning it absorbs moisture from the environment, which could potentially affect its stability and efficacy. It is recommended to store the compound in a cool, dry, and well-ventilated place .
Biochemical Analysis
Biochemical Properties
1,3-Bis(2,6-diisopropylphenyl)-4,5-dihydro-1H-imidazol-3-ium chloride plays a significant role in biochemical reactions as a ligand. It is used with palladium acetate to generate an N-heterocyclic carbene catalyst for carbonylative cross-coupling of pyridyl halides with aryl boronic acids . This compound interacts with enzymes and proteins involved in these reactions, facilitating the formation of carbon-carbon bonds. The nature of these interactions involves the stabilization of transition states and the activation of substrates, making the reactions more efficient.
Cellular Effects
The effects of this compound on various types of cells and cellular processes are profound. It influences cell function by affecting cell signaling pathways, gene expression, and cellular metabolism. This compound can modulate the activity of specific enzymes and proteins, leading to changes in metabolic flux and the levels of various metabolites
Molecular Mechanism
At the molecular level, this compound exerts its effects through binding interactions with biomolecules. It acts as a ligand, forming complexes with metal ions such as palladium, which are essential for catalytic activity . These complexes facilitate the activation of substrates and the stabilization of transition states, leading to efficient catalytic reactions. Additionally, this compound can influence gene expression by interacting with transcription factors and other regulatory proteins.
Temporal Effects in Laboratory Settings
In laboratory settings, the effects of this compound change over time. The compound exhibits good stability, but its activity can degrade under certain conditions Long-term studies have shown that it can have lasting effects on cellular function, particularly in in vitro and in vivo studies
Dosage Effects in Animal Models
The effects of this compound vary with different dosages in animal models. At lower doses, the compound can have beneficial effects, such as enhancing catalytic activity and improving metabolic processes . At higher doses, it can exhibit toxic or adverse effects, including enzyme inhibition and disruption of cellular functions. Understanding these dosage effects is crucial for determining the safe and effective use of this compound in various applications.
Metabolic Pathways
This compound is involved in several metabolic pathways. It interacts with enzymes and cofactors that are essential for its catalytic activity These interactions can affect metabolic flux and the levels of various metabolites, leading to changes in cellular metabolism
Transport and Distribution
Within cells and tissues, this compound is transported and distributed through interactions with transporters and binding proteins . These interactions can influence the compound’s localization and accumulation, affecting its activity and function. Understanding these transport and distribution mechanisms is essential for optimizing the compound’s use in various applications.
Subcellular Localization
The subcellular localization of this compound is crucial for its activity and function. The compound can be directed to specific compartments or organelles through targeting signals or post-translational modifications . These localization mechanisms are essential for understanding how the compound exerts its effects at the cellular level and optimizing its use in various applications.
Preparation Methods
Synthetic Routes and Reaction Conditions
1,3-Bis(2,6-diisopropylphenyl)-4,5-dihydro-1H-imidazol-3-ium chloride can be synthesized by reacting 1,3-bis(2,6-diisopropylphenyl)-1,3-dihydro-2H-imidazol-2-ylidene with silicon tetrachloride (SiCl4) in deuterated dichloromethane . The reaction typically takes place over two weeks at a temperature of 253 K, resulting in the formation of colorless needles of the compound .
Industrial Production Methods
Industrial production methods for this compound often involve similar synthetic routes but are optimized for larger-scale production. The use of palladium acetate to generate an N-heterocyclic carbene catalyst for carbonylative cross-coupling of pyridyl halides with aryl boronic acids is one such method .
Chemical Reactions Analysis
Types of Reactions
1,3-Bis(2,6-diisopropylphenyl)-4,5-dihydro-1H-imidazol-3-ium chloride undergoes several types of reactions, including:
Oxidation: This compound can be oxidized under specific conditions.
Reduction: It can also undergo reduction reactions.
Substitution: Commonly involved in substitution reactions, particularly in organic synthesis.
Common Reagents and Conditions
Oxidation: Typically involves oxidizing agents such as hydrogen peroxide or potassium permanganate.
Reduction: Reducing agents like lithium aluminum hydride or sodium borohydride are often used.
Substitution: Conditions vary depending on the specific substitution reaction but often involve catalysts like palladium acetate.
Major Products
The major products formed from these reactions depend on the specific reagents and conditions used. For example, the catalytic cycloisomerization of 2-(iodoethynyl)aryl esters using this compound results in the formation of 3-iodo-2-acyl benzofurans .
Scientific Research Applications
1,3-Bis(2,6-diisopropylphenyl)-4,5-dihydro-1H-imidazol-3-ium chloride has a wide range of scientific research applications:
Comparison with Similar Compounds
Similar Compounds
1,3-Bis(2,6-diisopropylphenyl)imidazol-2-ylidene (IPr): A similar compound with a slightly different structure but similar catalytic properties.
1,3-Bis(2,6-diisopropylphenyl)-4,5-dihydroimidazol-2-ylidene (SIPr): The saturated analogue of the compound, also used in catalysis.
1,3-Bis(2,6-diisopropylphenyl)imidazolium chloride (SIPr-HCl): Another closely related compound with similar applications.
Uniqueness
1,3-Bis(2,6-diisopropylphenyl)-4,5-dihydro-1H-imidazol-3-ium chloride is unique due to its excellent thermal stability, good solubility in organic solvents, and notable catalytic activity . These properties make it a valuable tool in organic synthesis and various industrial applications .
Properties
CAS No. |
258278-25-0 |
---|---|
Molecular Formula |
C27H39N2+ |
Molecular Weight |
391.6 g/mol |
IUPAC Name |
1,3-bis[2,6-di(propan-2-yl)phenyl]-4,5-dihydroimidazol-1-ium |
InChI |
InChI=1S/C27H39N2/c1-18(2)22-11-9-12-23(19(3)4)26(22)28-15-16-29(17-28)27-24(20(5)6)13-10-14-25(27)21(7)8/h9-14,17-21H,15-16H2,1-8H3/q+1 |
InChI Key |
SCEZRJLZOZKPBC-UHFFFAOYSA-N |
SMILES |
CC(C)C1=C(C(=CC=C1)C(C)C)N2CC[N+](=C2)C3=C(C=CC=C3C(C)C)C(C)C.[Cl-] |
Canonical SMILES |
CC(C)C1=C(C(=CC=C1)C(C)C)N2CC[N+](=C2)C3=C(C=CC=C3C(C)C)C(C)C |
Pictograms |
Irritant |
Origin of Product |
United States |
Retrosynthesis Analysis
AI-Powered Synthesis Planning: Our tool employs the Template_relevance Pistachio, Template_relevance Bkms_metabolic, Template_relevance Pistachio_ringbreaker, Template_relevance Reaxys, Template_relevance Reaxys_biocatalysis model, leveraging a vast database of chemical reactions to predict feasible synthetic routes.
One-Step Synthesis Focus: Specifically designed for one-step synthesis, it provides concise and direct routes for your target compounds, streamlining the synthesis process.
Accurate Predictions: Utilizing the extensive PISTACHIO, BKMS_METABOLIC, PISTACHIO_RINGBREAKER, REAXYS, REAXYS_BIOCATALYSIS database, our tool offers high-accuracy predictions, reflecting the latest in chemical research and data.
Strategy Settings
Precursor scoring | Relevance Heuristic |
---|---|
Min. plausibility | 0.01 |
Model | Template_relevance |
Template Set | Pistachio/Bkms_metabolic/Pistachio_ringbreaker/Reaxys/Reaxys_biocatalysis |
Top-N result to add to graph | 6 |
Feasible Synthetic Routes
Disclaimer and Information on In-Vitro Research Products
Please be aware that all articles and product information presented on BenchChem are intended solely for informational purposes. The products available for purchase on BenchChem are specifically designed for in-vitro studies, which are conducted outside of living organisms. In-vitro studies, derived from the Latin term "in glass," involve experiments performed in controlled laboratory settings using cells or tissues. It is important to note that these products are not categorized as medicines or drugs, and they have not received approval from the FDA for the prevention, treatment, or cure of any medical condition, ailment, or disease. We must emphasize that any form of bodily introduction of these products into humans or animals is strictly prohibited by law. It is essential to adhere to these guidelines to ensure compliance with legal and ethical standards in research and experimentation.