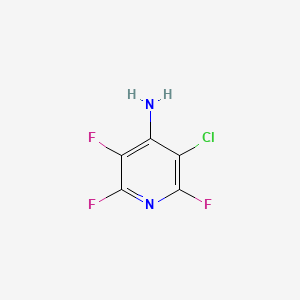
4-Amino-3-chloro-2,5,6-trifluoropyridine
Overview
Description
4-Amino-3-chloro-2,5,6-trifluoropyridine is a chemical compound with the molecular formula C5H2ClF3N2 and a molecular weight of 182.53 g/mol This compound is characterized by the presence of an amino group, a chlorine atom, and three fluorine atoms attached to a pyridine ring
Mechanism of Action
Target of Action
It is often used in proteomics research , suggesting that it may interact with proteins or enzymes in the body.
Biochemical Pathways
Given its use in proteomics research , it may be involved in protein synthesis or modification pathways.
Action Environment
Environmental factors can significantly influence the action, efficacy, and stability of a compound. Factors such as temperature, pH, and the presence of other molecules can affect the activity and stability of 4-Amino-3-chloro-2,5,6-trifluoropyridine . .
Biochemical Analysis
Biochemical Properties
4-Amino-3-chloro-2,5,6-trifluoropyridine plays a significant role in biochemical reactions. It interacts with various enzymes, proteins, and other biomolecules. For instance, it has been observed to interact with certain proteases and kinases, influencing their activity. The nature of these interactions often involves binding to the active sites of enzymes, thereby modulating their catalytic functions. This compound’s trifluoromethyl groups can enhance its binding affinity to hydrophobic pockets within proteins, making it a valuable tool in studying enzyme mechanisms and protein-ligand interactions .
Cellular Effects
The effects of this compound on cells and cellular processes are profound. It can influence cell signaling pathways, gene expression, and cellular metabolism. For example, it has been shown to modulate the activity of certain transcription factors, leading to changes in gene expression profiles. Additionally, this compound can affect cellular metabolism by interacting with metabolic enzymes, thereby altering the flux of metabolic pathways. These effects can vary depending on the cell type and the concentration of the compound used .
Molecular Mechanism
At the molecular level, this compound exerts its effects through several mechanisms. It can bind to specific biomolecules, such as enzymes and receptors, altering their conformation and activity. This binding can result in either inhibition or activation of the target molecules. For instance, it may inhibit the activity of certain kinases by occupying their ATP-binding sites, thereby preventing phosphorylation events essential for signal transduction. Additionally, this compound can influence gene expression by interacting with transcription factors and other regulatory proteins .
Temporal Effects in Laboratory Settings
In laboratory settings, the effects of this compound can change over time. The compound’s stability and degradation are critical factors that influence its long-term effects on cellular function. Studies have shown that it remains relatively stable under standard laboratory conditions, but its activity can diminish over extended periods due to gradual degradation. Long-term exposure to this compound in in vitro or in vivo studies has revealed sustained effects on cellular processes, although the specific outcomes can vary depending on the experimental conditions .
Dosage Effects in Animal Models
The effects of this compound in animal models are dose-dependent. At lower doses, it may exhibit minimal or no adverse effects, while higher doses can lead to toxicity. Threshold effects have been observed, where a certain concentration is required to elicit a measurable biological response. Toxic effects at high doses can include cellular damage, disruption of metabolic processes, and adverse physiological responses. These findings highlight the importance of careful dosage optimization in experimental studies involving this compound .
Metabolic Pathways
This compound is involved in various metabolic pathways. It interacts with enzymes and cofactors that facilitate its biotransformation and clearance from the body. The compound can undergo phase I and phase II metabolic reactions, including oxidation, reduction, and conjugation. These metabolic processes can influence the compound’s activity and toxicity, as well as its overall pharmacokinetic profile. Understanding these pathways is crucial for predicting the compound’s behavior in biological systems .
Transport and Distribution
The transport and distribution of this compound within cells and tissues are mediated by specific transporters and binding proteins. These interactions determine the compound’s localization and accumulation in different cellular compartments. For example, it may be actively transported into cells via membrane transporters or passively diffuse across cell membranes. Once inside the cell, it can bind to intracellular proteins, influencing its distribution and activity. These processes are essential for understanding the compound’s pharmacodynamics and therapeutic potential .
Preparation Methods
Synthetic Routes and Reaction Conditions: 4-Amino-3-chloro-2,5,6-trifluoropyridine can be synthesized from pentachloropyridine through a series of substitution reactions. The process involves the selective replacement of chlorine atoms with fluorine and amino groups under controlled conditions . The reaction typically requires the use of ammonia and fluorinating agents such as hydrogen fluoride or other fluorine sources.
Industrial Production Methods: In industrial settings, the production of this compound involves large-scale chemical reactors where the reaction conditions are optimized for maximum yield and purity. The process may include steps such as distillation, crystallization, and purification to ensure the final product meets the required specifications .
Chemical Reactions Analysis
Types of Reactions: 4-Amino-3-chloro-2,5,6-trifluoropyridine undergoes various chemical reactions, including:
Substitution Reactions: The chlorine and fluorine atoms can be replaced by other functional groups through nucleophilic or electrophilic substitution reactions.
Oxidation and Reduction: The compound can be oxidized or reduced under specific conditions to form different derivatives.
Coupling Reactions: It can participate in coupling reactions to form more complex molecules.
Common Reagents and Conditions:
Nucleophilic Substitution: Reagents such as sodium azide or potassium hydroxide can be used.
Oxidation: Oxidizing agents like potassium permanganate or hydrogen peroxide.
Reduction: Reducing agents such as lithium aluminum hydride or sodium borohydride.
Major Products Formed: The major products formed from these reactions depend on the specific reagents and conditions used. For example, nucleophilic substitution can yield azido derivatives, while oxidation can produce various oxidized forms of the compound .
Scientific Research Applications
4-Amino-3-chloro-2,5,6-trifluoropyridine has several applications in scientific research, including:
Chemistry: It is used as a building block for the synthesis of more complex organic molecules and fluorinated compounds.
Biology: The compound is studied for its potential biological activities and interactions with biomolecules.
Medicine: Research is ongoing to explore its potential as a pharmaceutical intermediate or active ingredient.
Industry: It is used in the development of agrochemicals, dyes, and other industrial products
Comparison with Similar Compounds
- 2-Amino-4-chloro-3,5,6-trifluoropyridine
- 5-Chloro-2,4,6-trifluoropyrimidine
Comparison: 4-Amino-3-chloro-2,5,6-trifluoropyridine is unique due to its specific substitution pattern on the pyridine ring, which imparts distinct chemical and physical properties. Compared to similar compounds, it may exhibit different reactivity, stability, and biological activity, making it suitable for specific applications .
Properties
IUPAC Name |
3-chloro-2,5,6-trifluoropyridin-4-amine | |
---|---|---|
Source | PubChem | |
URL | https://pubchem.ncbi.nlm.nih.gov | |
Description | Data deposited in or computed by PubChem | |
InChI |
InChI=1S/C5H2ClF3N2/c6-1-3(10)2(7)5(9)11-4(1)8/h(H2,10,11) | |
Source | PubChem | |
URL | https://pubchem.ncbi.nlm.nih.gov | |
Description | Data deposited in or computed by PubChem | |
InChI Key |
IJGMBUFHHBUVNG-UHFFFAOYSA-N | |
Source | PubChem | |
URL | https://pubchem.ncbi.nlm.nih.gov | |
Description | Data deposited in or computed by PubChem | |
Canonical SMILES |
C1(=C(C(=NC(=C1Cl)F)F)F)N | |
Source | PubChem | |
URL | https://pubchem.ncbi.nlm.nih.gov | |
Description | Data deposited in or computed by PubChem | |
Molecular Formula |
C5H2ClF3N2 | |
Source | PubChem | |
URL | https://pubchem.ncbi.nlm.nih.gov | |
Description | Data deposited in or computed by PubChem | |
DSSTOX Substance ID |
DTXSID00392952 | |
Record name | 4-Amino-3-chloro-2,5,6-trifluoropyridine | |
Source | EPA DSSTox | |
URL | https://comptox.epa.gov/dashboard/DTXSID00392952 | |
Description | DSSTox provides a high quality public chemistry resource for supporting improved predictive toxicology. | |
Molecular Weight |
182.53 g/mol | |
Source | PubChem | |
URL | https://pubchem.ncbi.nlm.nih.gov | |
Description | Data deposited in or computed by PubChem | |
CAS No. |
2693-57-4 | |
Record name | 4-Amino-3-chloro-2,5,6-trifluoropyridine | |
Source | EPA DSSTox | |
URL | https://comptox.epa.gov/dashboard/DTXSID00392952 | |
Description | DSSTox provides a high quality public chemistry resource for supporting improved predictive toxicology. | |
Record name | 4-Amino-3-chloro-2,5,6-trifluoro-pyridine | |
Source | European Chemicals Agency (ECHA) | |
URL | https://echa.europa.eu/information-on-chemicals | |
Description | The European Chemicals Agency (ECHA) is an agency of the European Union which is the driving force among regulatory authorities in implementing the EU's groundbreaking chemicals legislation for the benefit of human health and the environment as well as for innovation and competitiveness. | |
Explanation | Use of the information, documents and data from the ECHA website is subject to the terms and conditions of this Legal Notice, and subject to other binding limitations provided for under applicable law, the information, documents and data made available on the ECHA website may be reproduced, distributed and/or used, totally or in part, for non-commercial purposes provided that ECHA is acknowledged as the source: "Source: European Chemicals Agency, http://echa.europa.eu/". Such acknowledgement must be included in each copy of the material. ECHA permits and encourages organisations and individuals to create links to the ECHA website under the following cumulative conditions: Links can only be made to webpages that provide a link to the Legal Notice page. | |
Synthesis routes and methods I
Procedure details
Synthesis routes and methods II
Procedure details
Retrosynthesis Analysis
AI-Powered Synthesis Planning: Our tool employs the Template_relevance Pistachio, Template_relevance Bkms_metabolic, Template_relevance Pistachio_ringbreaker, Template_relevance Reaxys, Template_relevance Reaxys_biocatalysis model, leveraging a vast database of chemical reactions to predict feasible synthetic routes.
One-Step Synthesis Focus: Specifically designed for one-step synthesis, it provides concise and direct routes for your target compounds, streamlining the synthesis process.
Accurate Predictions: Utilizing the extensive PISTACHIO, BKMS_METABOLIC, PISTACHIO_RINGBREAKER, REAXYS, REAXYS_BIOCATALYSIS database, our tool offers high-accuracy predictions, reflecting the latest in chemical research and data.
Strategy Settings
Precursor scoring | Relevance Heuristic |
---|---|
Min. plausibility | 0.01 |
Model | Template_relevance |
Template Set | Pistachio/Bkms_metabolic/Pistachio_ringbreaker/Reaxys/Reaxys_biocatalysis |
Top-N result to add to graph | 6 |
Feasible Synthetic Routes
Disclaimer and Information on In-Vitro Research Products
Please be aware that all articles and product information presented on BenchChem are intended solely for informational purposes. The products available for purchase on BenchChem are specifically designed for in-vitro studies, which are conducted outside of living organisms. In-vitro studies, derived from the Latin term "in glass," involve experiments performed in controlled laboratory settings using cells or tissues. It is important to note that these products are not categorized as medicines or drugs, and they have not received approval from the FDA for the prevention, treatment, or cure of any medical condition, ailment, or disease. We must emphasize that any form of bodily introduction of these products into humans or animals is strictly prohibited by law. It is essential to adhere to these guidelines to ensure compliance with legal and ethical standards in research and experimentation.