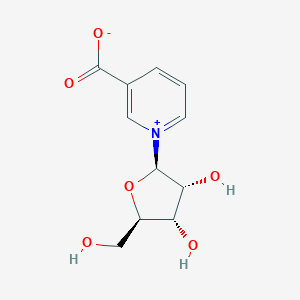
Nicotinic acid riboside
Overview
Description
Nicotinic acid riboside is a derivative of nicotinic acid, which is a form of vitamin B3. It is a pyridine-nucleoside and functions as a precursor to nicotinamide adenine dinucleotide (NAD+), a crucial coenzyme in various metabolic processes. This compound has gained attention for its potential health benefits, particularly in enhancing cellular energy metabolism and promoting longevity .
Mechanism of Action
Target of Action
Nicotinic acid riboside (NR) is a precursor of nicotinamide adenine dinucleotide (NAD+), an essential coenzyme that plays important roles in various metabolic pathways . The primary targets of NR are the enzymes involved in NAD+ biosynthesis and metabolism .
Mode of Action
NR is converted into NAD+ through a series of biochemical reactions. It first gets converted into nicotinamide mononucleotide (NMN) by the action of nicotinamide riboside kinases (NRKs). NMN is then converted into NAD+ by nicotinamide mononucleotide adenylyltransferases (NMNATs) . This conversion process allows NR to enhance the intracellular levels of NAD+, thereby influencing the activities of NAD±dependent enzymes .
Biochemical Pathways
NR is involved in the NAD+ biosynthetic pathway. It is converted into NMN by NRKs, and NMN is further converted into NAD+ by NMNATs . NAD+ is a crucial coenzyme in various metabolic redox reactions, and it also serves as a substrate for NAD±consuming enzymes, which are involved in many fundamental cellular processes including aging and longevity .
Pharmacokinetics
Oral administration of NR has been shown to effectively increase NAD+ levels in the body. In a clinical trial, single doses of 100, 300, and 1,000 mg of NR produced dose-dependent increases in the blood NAD+ metabolome . This suggests that NR has good bioavailability and can effectively enhance NAD+ levels in the body.
Result of Action
The increase in NAD+ levels due to NR administration has several molecular and cellular effects. It enhances the activities of NAD±dependent enzymes, which play key roles in various cellular processes such as metabolism, DNA repair, cell growth, and survival . This can have protective effects against a broad spectrum of pathological conditions, such as neurodegenerative diseases, diabetes, and hearing loss .
Biochemical Analysis
Biochemical Properties
NAR plays a significant role in biochemical reactions as it is converted within the body to NAD+, which is required for various critical cell functions, including metabolism, DNA repair, cell growth, and survival . NAR interacts with enzymes such as nicotinamide riboside kinases (NRK1, NRK2), which are involved in its conversion to NAD+ .
Cellular Effects
NAR has been shown to have a broad impact on various types of cells and cellular processes. It influences cell function by boosting intracellular NAD+ concentrations, which can provide protective effects against a range of pathological conditions, such as neurodegenerative diseases, diabetes, and hearing loss . It also impacts cell signaling pathways, gene expression, and cellular metabolism .
Molecular Mechanism
The molecular mechanism of NAR action involves its conversion to NAD+ through a two-step and a three-step pathway . This process includes binding interactions with biomolecules, enzyme activation, and changes in gene expression. The conversion of NAR to NAD+ is crucial for various metabolic reactions and cellular functions .
Temporal Effects in Laboratory Settings
The effects of NAR change over time in laboratory settings. NAR is readily metabolized, resulting in the generation of nicotinamide (Nam) after being imported into cells . Information on the product’s stability, degradation, and long-term effects on cellular function is still being researched.
Metabolic Pathways
NAR is involved in the NAD+ biosynthetic pathway. It is metabolized by two main pathways: degradative processes from which Nam results, and synthetic processes exploiting NR kinases (NRK1, NRK2), resulting in increased tissue NAD+ .
Transport and Distribution
NAR is transported into cells by members of the equilibrative nucleoside transporter (ENT) family ENT1, ENT2, and ENT4 . After being imported into cells, NAR is readily metabolized .
Subcellular Localization
As a precursor to NAD+, NAR is likely to be found wherever NAD+ is utilized within the cell, including the mitochondria .
Preparation Methods
Synthetic Routes and Reaction Conditions: Nicotinic acid riboside can be synthesized through several methods. One common approach involves the glycosylation of nicotinic acid with ribose. This reaction typically requires an acid catalyst and proceeds under mild conditions to yield this compound. Another method involves the enzymatic conversion of nicotinic acid mononucleotide to this compound using specific ribosyltransferases .
Industrial Production Methods: Industrial production of this compound often employs biotechnological processes. Microbial fermentation using genetically engineered strains of bacteria or yeast can produce this compound efficiently. These microorganisms are engineered to overexpress the enzymes required for the biosynthesis of this compound from simple sugars and nicotinic acid .
Chemical Reactions Analysis
Types of Reactions: Nicotinic acid riboside undergoes various chemical reactions, including:
Oxidation: It can be oxidized to form this compound-5’-phosphate.
Reduction: Reduction reactions can convert this compound to dihydrothis compound.
Substitution: It can participate in nucleophilic substitution reactions, where the ribose moiety is replaced by other sugar derivatives.
Common Reagents and Conditions:
Oxidation: Common oxidizing agents include potassium permanganate and hydrogen peroxide.
Reduction: Sodium borohydride and lithium aluminum hydride are frequently used reducing agents.
Substitution: Acidic or basic conditions can facilitate substitution reactions, often using catalysts like sulfuric acid or sodium hydroxide.
Major Products:
Oxidation: this compound-5’-phosphate.
Reduction: Dihydrothis compound.
Substitution: Various ribose derivatives depending on the substituent used.
Scientific Research Applications
Nicotinic acid riboside has a wide range of scientific research applications:
Chemistry: It is used as a precursor in the synthesis of NAD+ analogs and derivatives.
Biology: Studies have shown its role in enhancing cellular NAD+ levels, which is crucial for energy metabolism and DNA repair.
Medicine: It has potential therapeutic applications in treating metabolic disorders, neurodegenerative diseases, and age-related conditions.
Industry: this compound is used in the formulation of dietary supplements aimed at boosting NAD+ levels and improving overall health
Comparison with Similar Compounds
Nicotinamide riboside: Another NAD+ precursor with similar functions but different metabolic pathways.
Nicotinamide mononucleotide: A direct precursor to NAD+ that bypasses some of the enzymatic steps required for nicotinic acid riboside conversion.
Nicotinamide: A form of vitamin B3 that also contributes to NAD+ synthesis but has different pharmacological properties
Uniqueness: this compound is unique in its ability to efficiently increase NAD+ levels through both Nrk1-dependent and Nrk1-independent pathways. This dual pathway utilization makes it a versatile and potent NAD+ precursor compared to other similar compounds .
Properties
IUPAC Name |
1-[(2R,3R,4S,5R)-3,4-dihydroxy-5-(hydroxymethyl)oxolan-2-yl]pyridin-1-ium-3-carboxylate | |
---|---|---|
Source | PubChem | |
URL | https://pubchem.ncbi.nlm.nih.gov | |
Description | Data deposited in or computed by PubChem | |
InChI |
InChI=1S/C11H13NO6/c13-5-7-8(14)9(15)10(18-7)12-3-1-2-6(4-12)11(16)17/h1-4,7-10,13-15H,5H2/t7-,8-,9-,10-/m1/s1 | |
Source | PubChem | |
URL | https://pubchem.ncbi.nlm.nih.gov | |
Description | Data deposited in or computed by PubChem | |
InChI Key |
PUEDDPCUCPRQNY-ZYUZMQFOSA-N | |
Source | PubChem | |
URL | https://pubchem.ncbi.nlm.nih.gov | |
Description | Data deposited in or computed by PubChem | |
Canonical SMILES |
C1=CC(=C[N+](=C1)C2C(C(C(O2)CO)O)O)C(=O)[O-] | |
Source | PubChem | |
URL | https://pubchem.ncbi.nlm.nih.gov | |
Description | Data deposited in or computed by PubChem | |
Isomeric SMILES |
C1=CC(=C[N+](=C1)[C@H]2[C@@H]([C@@H]([C@H](O2)CO)O)O)C(=O)[O-] | |
Source | PubChem | |
URL | https://pubchem.ncbi.nlm.nih.gov | |
Description | Data deposited in or computed by PubChem | |
Molecular Formula |
C11H13NO6 | |
Source | PubChem | |
URL | https://pubchem.ncbi.nlm.nih.gov | |
Description | Data deposited in or computed by PubChem | |
DSSTOX Substance ID |
DTXSID20938912 | |
Record name | Nicotinic acid ribonucleoside | |
Source | EPA DSSTox | |
URL | https://comptox.epa.gov/dashboard/DTXSID20938912 | |
Description | DSSTox provides a high quality public chemistry resource for supporting improved predictive toxicology. | |
Molecular Weight |
255.22 g/mol | |
Source | PubChem | |
URL | https://pubchem.ncbi.nlm.nih.gov | |
Description | Data deposited in or computed by PubChem | |
CAS No. |
17720-18-2 | |
Record name | Pyridinium, 3-carboxy-1-β-D-ribofuranosyl-, inner salt | |
Source | CAS Common Chemistry | |
URL | https://commonchemistry.cas.org/detail?cas_rn=17720-18-2 | |
Description | CAS Common Chemistry is an open community resource for accessing chemical information. Nearly 500,000 chemical substances from CAS REGISTRY cover areas of community interest, including common and frequently regulated chemicals, and those relevant to high school and undergraduate chemistry classes. This chemical information, curated by our expert scientists, is provided in alignment with our mission as a division of the American Chemical Society. | |
Explanation | The data from CAS Common Chemistry is provided under a CC-BY-NC 4.0 license, unless otherwise stated. | |
Record name | Nicotinic acid ribonucleoside | |
Source | ChemIDplus | |
URL | https://pubchem.ncbi.nlm.nih.gov/substance/?source=chemidplus&sourceid=0017720182 | |
Description | ChemIDplus is a free, web search system that provides access to the structure and nomenclature authority files used for the identification of chemical substances cited in National Library of Medicine (NLM) databases, including the TOXNET system. | |
Record name | Nicotinic acid ribonucleoside | |
Source | EPA DSSTox | |
URL | https://comptox.epa.gov/dashboard/DTXSID20938912 | |
Description | DSSTox provides a high quality public chemistry resource for supporting improved predictive toxicology. | |
Retrosynthesis Analysis
AI-Powered Synthesis Planning: Our tool employs the Template_relevance Pistachio, Template_relevance Bkms_metabolic, Template_relevance Pistachio_ringbreaker, Template_relevance Reaxys, Template_relevance Reaxys_biocatalysis model, leveraging a vast database of chemical reactions to predict feasible synthetic routes.
One-Step Synthesis Focus: Specifically designed for one-step synthesis, it provides concise and direct routes for your target compounds, streamlining the synthesis process.
Accurate Predictions: Utilizing the extensive PISTACHIO, BKMS_METABOLIC, PISTACHIO_RINGBREAKER, REAXYS, REAXYS_BIOCATALYSIS database, our tool offers high-accuracy predictions, reflecting the latest in chemical research and data.
Strategy Settings
Precursor scoring | Relevance Heuristic |
---|---|
Min. plausibility | 0.01 |
Model | Template_relevance |
Template Set | Pistachio/Bkms_metabolic/Pistachio_ringbreaker/Reaxys/Reaxys_biocatalysis |
Top-N result to add to graph | 6 |
Feasible Synthetic Routes
Disclaimer and Information on In-Vitro Research Products
Please be aware that all articles and product information presented on BenchChem are intended solely for informational purposes. The products available for purchase on BenchChem are specifically designed for in-vitro studies, which are conducted outside of living organisms. In-vitro studies, derived from the Latin term "in glass," involve experiments performed in controlled laboratory settings using cells or tissues. It is important to note that these products are not categorized as medicines or drugs, and they have not received approval from the FDA for the prevention, treatment, or cure of any medical condition, ailment, or disease. We must emphasize that any form of bodily introduction of these products into humans or animals is strictly prohibited by law. It is essential to adhere to these guidelines to ensure compliance with legal and ethical standards in research and experimentation.