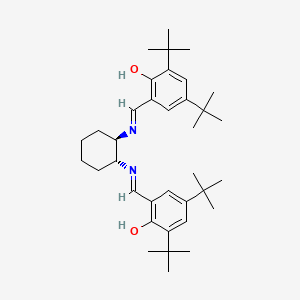
(R,R)-(-)-N,N'-Bis(3,5-di-tert-butylsalicylidene)-1,2-cyclohexanediamine
- Click on QUICK INQUIRY to receive a quote from our team of experts.
- With the quality product at a COMPETITIVE price, you can focus more on your research.
Overview
Description
(R,R)-(-)-N,N’-Bis(3,5-di-tert-butylsalicylidene)-1,2-cyclohexanediamine is a chiral salen ligand widely used in asymmetric catalysis. This compound is known for its ability to form stable complexes with various metals, making it a valuable tool in enantioselective synthesis. The presence of bulky tert-butyl groups enhances the steric hindrance around the metal center, improving the selectivity of catalytic reactions.
Mechanism of Action
Target of Action
It’s known that similar compounds have antioxidant properties , suggesting that they may interact with reactive oxygen species or enzymes involved in oxidative stress.
Mode of Action
It’s known that 3,5-di-tert-butyl-4-hydroxybenzaldehyde, a related compound, rearranges to various esters when oxidized with potassium ferricyanide in an alkaline medium . This suggests that the compound may undergo similar oxidative rearrangements, potentially leading to changes in its structure and function.
Action Environment
Environmental factors such as pH, temperature, and the presence of other reactive species could influence the compound’s action, efficacy, and stability. For example, the compound’s oxidative rearrangements occur in an alkaline medium , suggesting that pH could affect its reactivity. Similarly, temperature could influence the rate of these reactions, while other reactive species could compete with the compound for reactive oxygen species, potentially affecting its antioxidant efficacy.
Preparation Methods
Synthetic Routes and Reaction Conditions
The synthesis of (R,R)-(-)-N,N’-Bis(3,5-di-tert-butylsalicylidene)-1,2-cyclohexanediamine typically involves the condensation of 3,5-di-tert-butylsalicylaldehyde with (R,R)-1,2-diaminocyclohexane. The reaction is carried out in an organic solvent such as ethanol or methanol under reflux conditions. The resulting Schiff base is then purified by recrystallization.
Industrial Production Methods
Industrial production of this compound follows similar synthetic routes but on a larger scale. The process involves the use of high-purity starting materials and optimized reaction conditions to ensure high yield and purity of the final product. Advanced purification techniques such as column chromatography and crystallization are employed to obtain the desired compound.
Chemical Reactions Analysis
Types of Reactions
(R,R)-(-)-N,N’-Bis(3,5-di-tert-butylsalicylidene)-1,2-cyclohexanediamine undergoes various types of reactions, including:
Oxidation: The compound can be oxidized to form corresponding oxo-metal complexes.
Reduction: Reduction reactions can lead to the formation of reduced metal-salen complexes.
Substitution: The ligand can undergo substitution reactions where the metal center is replaced by another metal.
Common Reagents and Conditions
Oxidation: Common oxidizing agents include hydrogen peroxide and tert-butyl hydroperoxide.
Reduction: Reducing agents such as sodium borohydride and lithium aluminum hydride are used.
Substitution: Metal salts like copper(II) acetate and cobalt(II) chloride are commonly used in substitution reactions.
Major Products
The major products formed from these reactions are metal-salen complexes, which are used in various catalytic applications.
Scientific Research Applications
(R,R)-(-)-N,N’-Bis(3,5-di-tert-butylsalicylidene)-1,2-cyclohexanediamine has a wide range of applications in scientific research:
Chemistry: It is used as a ligand in asymmetric catalysis, particularly in the synthesis of chiral epoxides and diols.
Biology: The compound is studied for its potential in enzyme mimetics and as a model for metalloenzymes.
Medicine: Research is ongoing to explore its potential as a therapeutic agent in the treatment of diseases involving metal ion imbalance.
Industry: It is used in the production of fine chemicals and pharmaceuticals due to its ability to catalyze enantioselective reactions.
Comparison with Similar Compounds
Similar Compounds
- (S,S)-(+)-N,N’-Bis(3,5-di-tert-butylsalicylidene)-1,2-cyclohexanediamine
- (R,R)-N,N’-Bis(3,5-di-tert-butylsalicylidene)-1,2-cyclohexanediaminoaluminum chloride
- (R,R)-N,N’-Bis(3,5-di-tert-butylsalicylidene)-1,2-cyclohexanediaminomanganese(III) chloride
Uniqueness
(R,R)-(-)-N,N’-Bis(3,5-di-tert-butylsalicylidene)-1,2-cyclohexanediamine is unique due to its high enantioselectivity and stability in catalytic reactions. The presence of bulky tert-butyl groups enhances its performance compared to other similar compounds. Its ability to form stable complexes with a wide range of metals makes it a versatile ligand in asymmetric catalysis.
Properties
IUPAC Name |
2,4-ditert-butyl-6-[[(1R,2R)-2-[(3,5-ditert-butyl-2-hydroxyphenyl)methylideneamino]cyclohexyl]iminomethyl]phenol |
Source
|
---|---|---|
Source | PubChem | |
URL | https://pubchem.ncbi.nlm.nih.gov | |
Description | Data deposited in or computed by PubChem | |
InChI |
InChI=1S/C36H54N2O2/c1-33(2,3)25-17-23(31(39)27(19-25)35(7,8)9)21-37-29-15-13-14-16-30(29)38-22-24-18-26(34(4,5)6)20-28(32(24)40)36(10,11)12/h17-22,29-30,39-40H,13-16H2,1-12H3/t29-,30-/m1/s1 |
Source
|
Source | PubChem | |
URL | https://pubchem.ncbi.nlm.nih.gov | |
Description | Data deposited in or computed by PubChem | |
InChI Key |
FYNXDGNCEBQLGC-LOYHVIPDSA-N |
Source
|
Source | PubChem | |
URL | https://pubchem.ncbi.nlm.nih.gov | |
Description | Data deposited in or computed by PubChem | |
Canonical SMILES |
CC(C)(C)C1=CC(=C(C(=C1)C(C)(C)C)O)C=NC2CCCCC2N=CC3=C(C(=CC(=C3)C(C)(C)C)C(C)(C)C)O |
Source
|
Source | PubChem | |
URL | https://pubchem.ncbi.nlm.nih.gov | |
Description | Data deposited in or computed by PubChem | |
Isomeric SMILES |
CC(C)(C)C1=CC(=C(C(=C1)C(C)(C)C)O)C=N[C@@H]2CCCC[C@H]2N=CC3=C(C(=CC(=C3)C(C)(C)C)C(C)(C)C)O |
Source
|
Source | PubChem | |
URL | https://pubchem.ncbi.nlm.nih.gov | |
Description | Data deposited in or computed by PubChem | |
Molecular Formula |
C36H54N2O2 |
Source
|
Source | PubChem | |
URL | https://pubchem.ncbi.nlm.nih.gov | |
Description | Data deposited in or computed by PubChem | |
DSSTOX Substance ID |
DTXSID101113716 |
Source
|
Record name | Phenol, 2,2′-[(1R,2R)-1,2-cyclohexanediylbis[(E)-nitrilomethylidyne]]bis[4,6-bis(1,1-dimethylethyl)- | |
Source | EPA DSSTox | |
URL | https://comptox.epa.gov/dashboard/DTXSID101113716 | |
Description | DSSTox provides a high quality public chemistry resource for supporting improved predictive toxicology. | |
Molecular Weight |
546.8 g/mol |
Source
|
Source | PubChem | |
URL | https://pubchem.ncbi.nlm.nih.gov | |
Description | Data deposited in or computed by PubChem | |
CAS No. |
135616-40-9 |
Source
|
Record name | Phenol, 2,2′-[(1R,2R)-1,2-cyclohexanediylbis[(E)-nitrilomethylidyne]]bis[4,6-bis(1,1-dimethylethyl)- | |
Source | EPA DSSTox | |
URL | https://comptox.epa.gov/dashboard/DTXSID101113716 | |
Description | DSSTox provides a high quality public chemistry resource for supporting improved predictive toxicology. | |
Disclaimer and Information on In-Vitro Research Products
Please be aware that all articles and product information presented on BenchChem are intended solely for informational purposes. The products available for purchase on BenchChem are specifically designed for in-vitro studies, which are conducted outside of living organisms. In-vitro studies, derived from the Latin term "in glass," involve experiments performed in controlled laboratory settings using cells or tissues. It is important to note that these products are not categorized as medicines or drugs, and they have not received approval from the FDA for the prevention, treatment, or cure of any medical condition, ailment, or disease. We must emphasize that any form of bodily introduction of these products into humans or animals is strictly prohibited by law. It is essential to adhere to these guidelines to ensure compliance with legal and ethical standards in research and experimentation.