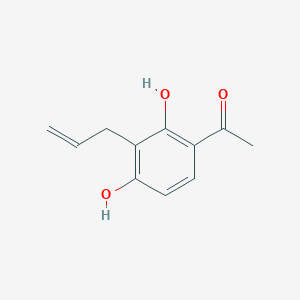
1-(3-Allyl-2,4-dihydroxyphenyl)ethanone
Overview
Description
1-(3-Allyl-2,4-dihydroxyphenyl)ethanone is an organic compound with the molecular formula C11H12O3 and a molecular weight of 192.21 g/mol It is a derivative of acetophenone, featuring hydroxyl groups at the 2 and 4 positions and an allyl group at the 3 position on the phenyl ring
Preparation Methods
Synthetic Routes and Reaction Conditions
1-(3-Allyl-2,4-dihydroxyphenyl)ethanone can be synthesized through several methods. One common approach involves the acetylation of 3-allylresorcinol using acetic anhydride in the presence of a catalyst such as zinc chloride . The reaction typically occurs under reflux conditions, and the product is purified through recrystallization.
Industrial Production Methods
Industrial production methods for this compound are not well-documented, but they likely involve similar acetylation reactions on a larger scale. The choice of solvents, catalysts, and purification techniques may vary to optimize yield and purity for industrial applications.
Chemical Reactions Analysis
Types of Reactions
1-(3-Allyl-2,4-dihydroxyphenyl)ethanone undergoes various chemical reactions, including:
Oxidation: The hydroxyl groups can be oxidized to form quinones.
Reduction: The carbonyl group can be reduced to form alcohols.
Substitution: The allyl group can participate in electrophilic substitution reactions.
Common Reagents and Conditions
Oxidation: Reagents such as potassium permanganate or chromium trioxide can be used under acidic conditions.
Reduction: Sodium borohydride or lithium aluminum hydride are common reducing agents.
Substitution: Electrophilic reagents like bromine or chlorine can be used for halogenation reactions.
Major Products
Oxidation: Quinones
Reduction: Alcohols
Substitution: Halogenated derivatives
Scientific Research Applications
1-(3-Allyl-2,4-dihydroxyphenyl)ethanone has several scientific research applications:
Chemistry: It is used as a building block in organic synthesis for the preparation of more complex molecules.
Biology: The compound’s hydroxyl groups make it a potential candidate for studying enzyme interactions and inhibition.
Medicine: It may have potential therapeutic applications due to its structural similarity to bioactive compounds.
Industry: It can be used in the synthesis of fine chemicals and pharmaceuticals.
Mechanism of Action
The mechanism of action of 1-(3-Allyl-2,4-dihydroxyphenyl)ethanone is not well-documented. its hydroxyl groups and carbonyl functionality suggest that it can interact with various biological targets, including enzymes and receptors. The compound may exert its effects through hydrogen bonding, hydrophobic interactions, and covalent modifications of target molecules.
Comparison with Similar Compounds
Similar Compounds
2,4-Dihydroxyacetophenone: Similar structure but lacks the allyl group.
1-(2,4-Dihydroxy-3-propylphenyl)ethanone: Similar structure with a propyl group instead of an allyl group.
2’,4’,6’-Trihydroxyacetophenone: Contains additional hydroxyl groups.
Uniqueness
1-(3-Allyl-2,4-dihydroxyphenyl)ethanone is unique due to the presence of the allyl group at the 3 position, which can participate in various chemical reactions, making it a versatile intermediate in organic synthesis .
Properties
IUPAC Name |
1-(2,4-dihydroxy-3-prop-2-enylphenyl)ethanone | |
---|---|---|
Source | PubChem | |
URL | https://pubchem.ncbi.nlm.nih.gov | |
Description | Data deposited in or computed by PubChem | |
InChI |
InChI=1S/C11H12O3/c1-3-4-9-10(13)6-5-8(7(2)12)11(9)14/h3,5-6,13-14H,1,4H2,2H3 | |
Source | PubChem | |
URL | https://pubchem.ncbi.nlm.nih.gov | |
Description | Data deposited in or computed by PubChem | |
InChI Key |
SCTZFHGSNSIMHU-UHFFFAOYSA-N | |
Source | PubChem | |
URL | https://pubchem.ncbi.nlm.nih.gov | |
Description | Data deposited in or computed by PubChem | |
Canonical SMILES |
CC(=O)C1=C(C(=C(C=C1)O)CC=C)O | |
Source | PubChem | |
URL | https://pubchem.ncbi.nlm.nih.gov | |
Description | Data deposited in or computed by PubChem | |
Molecular Formula |
C11H12O3 | |
Source | PubChem | |
URL | https://pubchem.ncbi.nlm.nih.gov | |
Description | Data deposited in or computed by PubChem | |
DSSTOX Substance ID |
DTXSID50379435 | |
Record name | 1-[2,4-Dihydroxy-3-(prop-2-en-1-yl)phenyl]ethan-1-one | |
Source | EPA DSSTox | |
URL | https://comptox.epa.gov/dashboard/DTXSID50379435 | |
Description | DSSTox provides a high quality public chemistry resource for supporting improved predictive toxicology. | |
Molecular Weight |
192.21 g/mol | |
Source | PubChem | |
URL | https://pubchem.ncbi.nlm.nih.gov | |
Description | Data deposited in or computed by PubChem | |
CAS No. |
38987-00-7 | |
Record name | 1-[2,4-Dihydroxy-3-(prop-2-en-1-yl)phenyl]ethan-1-one | |
Source | EPA DSSTox | |
URL | https://comptox.epa.gov/dashboard/DTXSID50379435 | |
Description | DSSTox provides a high quality public chemistry resource for supporting improved predictive toxicology. | |
Retrosynthesis Analysis
AI-Powered Synthesis Planning: Our tool employs the Template_relevance Pistachio, Template_relevance Bkms_metabolic, Template_relevance Pistachio_ringbreaker, Template_relevance Reaxys, Template_relevance Reaxys_biocatalysis model, leveraging a vast database of chemical reactions to predict feasible synthetic routes.
One-Step Synthesis Focus: Specifically designed for one-step synthesis, it provides concise and direct routes for your target compounds, streamlining the synthesis process.
Accurate Predictions: Utilizing the extensive PISTACHIO, BKMS_METABOLIC, PISTACHIO_RINGBREAKER, REAXYS, REAXYS_BIOCATALYSIS database, our tool offers high-accuracy predictions, reflecting the latest in chemical research and data.
Strategy Settings
Precursor scoring | Relevance Heuristic |
---|---|
Min. plausibility | 0.01 |
Model | Template_relevance |
Template Set | Pistachio/Bkms_metabolic/Pistachio_ringbreaker/Reaxys/Reaxys_biocatalysis |
Top-N result to add to graph | 6 |
Feasible Synthetic Routes
Disclaimer and Information on In-Vitro Research Products
Please be aware that all articles and product information presented on BenchChem are intended solely for informational purposes. The products available for purchase on BenchChem are specifically designed for in-vitro studies, which are conducted outside of living organisms. In-vitro studies, derived from the Latin term "in glass," involve experiments performed in controlled laboratory settings using cells or tissues. It is important to note that these products are not categorized as medicines or drugs, and they have not received approval from the FDA for the prevention, treatment, or cure of any medical condition, ailment, or disease. We must emphasize that any form of bodily introduction of these products into humans or animals is strictly prohibited by law. It is essential to adhere to these guidelines to ensure compliance with legal and ethical standards in research and experimentation.