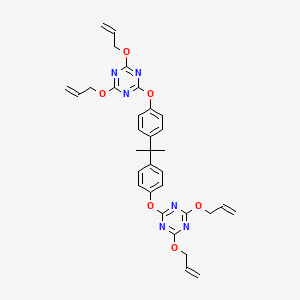
2,2'-(Isopropylidenebis(p-phenyleneoxy))bis(4,6-bis(allyloxy)-1,3,5-triazine)
- Click on QUICK INQUIRY to receive a quote from our team of experts.
- With the quality product at a COMPETITIVE price, you can focus more on your research.
Overview
Description
2,2’-[(Isopropylidenebis(p-phenyleneoxy)]bis[4,6-bis(allyloxy)-1,3,5-triazine] is a complex organic compound known for its unique structural properties and potential applications in various scientific fields. This compound features a triazine core with allyloxy groups and isopropylidene-linked phenyleneoxy groups, making it a versatile molecule for research and industrial applications.
Preparation Methods
The synthesis of 2,2’-[(Isopropylidenebis(p-phenyleneoxy)]bis[4,6-bis(allyloxy)-1,3,5-triazine] typically involves multiple steps, including the preparation of intermediate compounds and their subsequent reactions under controlled conditions. One common method involves the reaction of 4,6-dichloro-1,3,5-triazine with allyl alcohol to form 4,6-bis(allyloxy)-1,3,5-triazine. This intermediate is then reacted with isopropylidenebis(p-phenyleneoxy) to yield the final product. Industrial production methods may involve optimization of reaction conditions, such as temperature, pressure, and catalysts, to enhance yield and purity .
Chemical Reactions Analysis
2,2’-[(Isopropylidenebis(p-phenyleneoxy)]bis[4,6-bis(allyloxy)-1,3,5-triazine] undergoes various chemical reactions, including:
Oxidation: The allyloxy groups can be oxidized to form epoxides or other oxygen-containing functional groups.
Reduction: The triazine ring can be reduced under specific conditions to form amine derivatives.
Substitution: The allyloxy groups can be substituted with other nucleophiles, such as halides or amines, to form new derivatives. Common reagents used in these reactions include oxidizing agents like hydrogen peroxide, reducing agents like lithium aluminum hydride, and nucleophiles like sodium azide
Scientific Research Applications
2,2’-[(Isopropylidenebis(p-phenyleneoxy)]bis[4,6-bis(allyloxy)-1,3,5-triazine] has a wide range of applications in scientific research:
Chemistry: It is used as a building block for the synthesis of advanced materials, including polymers and resins.
Biology: Its derivatives are studied for their potential biological activities, such as antimicrobial and anticancer properties.
Medicine: Research is ongoing to explore its use in drug delivery systems and as a component of therapeutic agents.
Industry: It is used in the production of high-performance coatings, adhesives, and sealants due to its thermal stability and chemical resistance
Mechanism of Action
The mechanism of action of 2,2’-[(Isopropylidenebis(p-phenyleneoxy)]bis[4,6-bis(allyloxy)-1,3,5-triazine] involves its interaction with molecular targets through its functional groups. The allyloxy groups can form covalent bonds with nucleophilic sites on target molecules, while the triazine core provides stability and rigidity. This compound can also participate in hydrogen bonding and π-π interactions, enhancing its binding affinity and specificity .
Comparison with Similar Compounds
Compared to other similar compounds, 2,2’-[(Isopropylidenebis(p-phenyleneoxy)]bis[4,6-bis(allyloxy)-1,3,5-triazine] stands out due to its unique combination of functional groups and structural features. Similar compounds include:
2,2-Bis[4-(4-aminophenoxy)phenyl]propane (BAPP): A diamino monomer used in the synthesis of polyimides.
4,4’-Isopropylidenebis(p-phenyleneoxy)dianiline (HFBAPP): Used in the preparation of epoxy resins with high thermal stability.
1,1’-Isopropylidenebis(p-phenyleneoxy)di-2-propanol diacrylate: A compound with similar structural elements but different functional groups
These compounds share some structural similarities but differ in their functional groups and specific applications, highlighting the versatility and uniqueness of 2,2’-[(Isopropylidenebis(p-phenyleneoxy)]bis[4,6-bis(allyloxy)-1,3,5-triazine].
Biological Activity
2,2'-(Isopropylidenebis(p-phenyleneoxy))bis(4,6-bis(allyloxy)-1,3,5-triazine) is a synthetic compound with potential biological applications. Its structure incorporates the s-triazine core, which is known for its diverse biological activities, including anticancer properties. This article reviews the biological activity of this compound, summarizing key research findings and case studies.
Chemical Structure and Properties
The compound has the following molecular formula: C33H34N6O6 and a molecular weight of 610.7 g/mol . The presence of the s-triazine core enhances its biological activity by allowing for modifications that can lead to various pharmacological effects.
Biological Activity Overview
Research indicates that derivatives of s-triazines exhibit a broad spectrum of biological activities:
- Anticancer Activity : Many s-triazine derivatives have shown significant inhibitory effects on cancer cell proliferation and induction of apoptosis. The mechanism often involves the inhibition of enzymes related to tumorigenesis and cell cycle regulation .
- Antioxidant Properties : Compounds with similar structures have demonstrated antioxidant capabilities by scavenging free radicals and reducing oxidative stress in cells .
- Anti-inflammatory Effects : Some derivatives exhibit anti-inflammatory properties by inhibiting pro-inflammatory enzymes such as lipoxygenase .
Anticancer Activity
A study highlighted that the compound's analogs were tested against various cancer cell lines, showing IC50 values indicating effective cytotoxicity:
- HeLa Cells : IC50 = 39.7 µM
- MCF-7 Cells : IC50 = 41.5 µM
- HL-60 Cells : IC50 = 23.1 µM
- HepG2 Cells : IC50 = 31.2 µM .
These findings suggest that modifications to the triazine structure can enhance potency against specific cancer types.
Antioxidant Activity
In vitro assays such as DPPH and ORAC were performed to evaluate the antioxidant capacity of similar compounds. Results indicated that certain derivatives could effectively neutralize free radicals, showcasing their potential as therapeutic agents against oxidative stress-related diseases .
Anti-inflammatory Activity
The compound's ability to inhibit 5-lipoxygenase was assessed, revealing significant inhibitory action with IC50 values in the sub-micromolar range. This suggests that it may be beneficial in treating inflammatory conditions .
Case Studies
- Photoprotective and Antioxidant Study : A series of related compounds were synthesized and tested for photoprotective properties. One derivative was found to be an excellent UVA filter while demonstrating notable antioxidant activity against various radical species .
- Multifunctional Applications : Research has indicated that compounds similar to 2,2'-(Isopropylidenebis(p-phenyleneoxy))bis(4,6-bis(allyloxy)-1,3,5-triazine) can be designed to target multiple biological pathways simultaneously, potentially simplifying treatment regimens in clinical settings .
Properties
CAS No. |
85896-29-3 |
---|---|
Molecular Formula |
C33H34N6O6 |
Molecular Weight |
610.7 g/mol |
IUPAC Name |
2-[4-[2-[4-[[4,6-bis(prop-2-enoxy)-1,3,5-triazin-2-yl]oxy]phenyl]propan-2-yl]phenoxy]-4,6-bis(prop-2-enoxy)-1,3,5-triazine |
InChI |
InChI=1S/C33H34N6O6/c1-7-19-40-27-34-28(41-20-8-2)37-31(36-27)44-25-15-11-23(12-16-25)33(5,6)24-13-17-26(18-14-24)45-32-38-29(42-21-9-3)35-30(39-32)43-22-10-4/h7-18H,1-4,19-22H2,5-6H3 |
InChI Key |
VQGHUYYABGMTQD-UHFFFAOYSA-N |
Canonical SMILES |
CC(C)(C1=CC=C(C=C1)OC2=NC(=NC(=N2)OCC=C)OCC=C)C3=CC=C(C=C3)OC4=NC(=NC(=N4)OCC=C)OCC=C |
Origin of Product |
United States |
Disclaimer and Information on In-Vitro Research Products
Please be aware that all articles and product information presented on BenchChem are intended solely for informational purposes. The products available for purchase on BenchChem are specifically designed for in-vitro studies, which are conducted outside of living organisms. In-vitro studies, derived from the Latin term "in glass," involve experiments performed in controlled laboratory settings using cells or tissues. It is important to note that these products are not categorized as medicines or drugs, and they have not received approval from the FDA for the prevention, treatment, or cure of any medical condition, ailment, or disease. We must emphasize that any form of bodily introduction of these products into humans or animals is strictly prohibited by law. It is essential to adhere to these guidelines to ensure compliance with legal and ethical standards in research and experimentation.