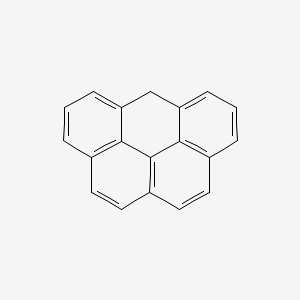
Olympicene
Overview
Description
Olympicene is an organic carbon-based molecule composed of five rings, four of which are benzene rings, arranged in the shape of the Olympic rings. The molecule was conceived in March 2010 by Graham Richards of the University of Oxford and Antony Williams as a way to celebrate the 2012 London Olympics . This compound has the chemical formula C19H12 and is a polycyclic aromatic hydrocarbon .
Preparation Methods
Synthetic Routes and Reaction Conditions: Olympicene can be synthesized through a series of organic reactions. One notable method involves a Wittig reaction of pyrene carboxaldehyde. The ylide needed for this reaction is prepared by reacting triphenyl phosphine with ethyl bromoacetate to form a phosphonium salt, which is then treated with a mild base . Another method involves a one-pot triply borylation-based double-fold borocyclization reaction to create boron-doped olympicenes .
Industrial Production Methods: While there is no large-scale industrial production of this compound, the synthetic methods developed in research laboratories provide a foundation for potential future applications in various industries .
Chemical Reactions Analysis
Types of Reactions: Olympicene undergoes various chemical reactions, including oxidation, reduction, and substitution reactions. These reactions are facilitated by the presence of multiple benzene rings, which provide reactive sites for different reagents .
Common Reagents and Conditions:
Oxidation: Common oxidizing agents like potassium permanganate or chromium trioxide can be used to oxidize this compound.
Reduction: Reducing agents such as lithium aluminum hydride or sodium borohydride can be employed to reduce this compound.
Substitution: Electrophilic aromatic substitution reactions can occur with reagents like bromine or chlorine under appropriate conditions.
Major Products: The major products formed from these reactions depend on the specific reagents and conditions used. For example, oxidation can lead to the formation of quinones, while reduction can yield partially hydrogenated derivatives .
Scientific Research Applications
Mechanism of Action
The mechanism by which olympicene exerts its effects is primarily related to its aromatic structure and electronic properties. The molecule’s planar structure and conjugated π-electron system allow it to interact with various molecular targets, including enzymes and receptors. These interactions can modulate biological pathways and influence cellular processes .
Comparison with Similar Compounds
Benzo[c]phenanthrene: Lacks the -CH2- spacer between the two sides of the molecule and has been studied for its electronic properties.
Naphthanthrone: Contains a ketone (C=O) group instead of the -CH2- spacer and has been known for decades.
Sulfur and Oxygen Analogues: Molecules where the -CH2- spacer has been replaced with sulfur or oxygen atoms, affecting their electronic properties.
Uniqueness: Olympicene’s unique structure, resembling the Olympic rings, and its combination of benzene rings make it distinct from other polycyclic aromatic hydrocarbons.
Properties
IUPAC Name |
pentacyclo[13.3.1.05,18.08,17.011,16]nonadeca-1,3,5(18),6,8(17),9,11(16),12,14-nonaene | |
---|---|---|
Details | Computed by Lexichem TK 2.7.0 (PubChem release 2021.10.14) | |
Source | PubChem | |
URL | https://pubchem.ncbi.nlm.nih.gov | |
Description | Data deposited in or computed by PubChem | |
InChI |
InChI=1S/C19H12/c1-3-12-7-9-14-10-8-13-4-2-6-16-11-15(5-1)17(12)19(14)18(13)16/h1-10H,11H2 | |
Details | Computed by InChI 1.0.6 (PubChem release 2021.10.14) | |
Source | PubChem | |
URL | https://pubchem.ncbi.nlm.nih.gov | |
Description | Data deposited in or computed by PubChem | |
InChI Key |
CFXWAPSYPXUYCO-UHFFFAOYSA-N | |
Details | Computed by InChI 1.0.6 (PubChem release 2021.10.14) | |
Source | PubChem | |
URL | https://pubchem.ncbi.nlm.nih.gov | |
Description | Data deposited in or computed by PubChem | |
Canonical SMILES |
C1C2=CC=CC3=C2C4=C(C=C3)C=CC5=C4C1=CC=C5 | |
Details | Computed by OEChem 2.3.0 (PubChem release 2021.10.14) | |
Source | PubChem | |
URL | https://pubchem.ncbi.nlm.nih.gov | |
Description | Data deposited in or computed by PubChem | |
Molecular Formula |
C19H12 | |
Details | Computed by PubChem 2.2 (PubChem release 2021.10.14) | |
Source | PubChem | |
URL | https://pubchem.ncbi.nlm.nih.gov | |
Description | Data deposited in or computed by PubChem | |
DSSTOX Substance ID |
DTXSID501018726 | |
Record name | Olympicene | |
Source | EPA DSSTox | |
URL | https://comptox.epa.gov/dashboard/DTXSID501018726 | |
Description | DSSTox provides a high quality public chemistry resource for supporting improved predictive toxicology. | |
Molecular Weight |
240.3 g/mol | |
Details | Computed by PubChem 2.2 (PubChem release 2021.10.14) | |
Source | PubChem | |
URL | https://pubchem.ncbi.nlm.nih.gov | |
Description | Data deposited in or computed by PubChem | |
CAS No. |
191-33-3 | |
Record name | 6H-Benzo[cd]pyrene | |
Source | CAS Common Chemistry | |
URL | https://commonchemistry.cas.org/detail?cas_rn=191-33-3 | |
Description | CAS Common Chemistry is an open community resource for accessing chemical information. Nearly 500,000 chemical substances from CAS REGISTRY cover areas of community interest, including common and frequently regulated chemicals, and those relevant to high school and undergraduate chemistry classes. This chemical information, curated by our expert scientists, is provided in alignment with our mission as a division of the American Chemical Society. | |
Explanation | The data from CAS Common Chemistry is provided under a CC-BY-NC 4.0 license, unless otherwise stated. | |
Record name | Olympicene | |
Source | ChemIDplus | |
URL | https://pubchem.ncbi.nlm.nih.gov/substance/?source=chemidplus&sourceid=0000191333 | |
Description | ChemIDplus is a free, web search system that provides access to the structure and nomenclature authority files used for the identification of chemical substances cited in National Library of Medicine (NLM) databases, including the TOXNET system. | |
Record name | Olympicene | |
Source | EPA DSSTox | |
URL | https://comptox.epa.gov/dashboard/DTXSID501018726 | |
Description | DSSTox provides a high quality public chemistry resource for supporting improved predictive toxicology. | |
Record name | OLYMPICENE | |
Source | FDA Global Substance Registration System (GSRS) | |
URL | https://gsrs.ncats.nih.gov/ginas/app/beta/substances/4R5BNS9ZCA | |
Description | The FDA Global Substance Registration System (GSRS) enables the efficient and accurate exchange of information on what substances are in regulated products. Instead of relying on names, which vary across regulatory domains, countries, and regions, the GSRS knowledge base makes it possible for substances to be defined by standardized, scientific descriptions. | |
Explanation | Unless otherwise noted, the contents of the FDA website (www.fda.gov), both text and graphics, are not copyrighted. They are in the public domain and may be republished, reprinted and otherwise used freely by anyone without the need to obtain permission from FDA. Credit to the U.S. Food and Drug Administration as the source is appreciated but not required. | |
Disclaimer and Information on In-Vitro Research Products
Please be aware that all articles and product information presented on BenchChem are intended solely for informational purposes. The products available for purchase on BenchChem are specifically designed for in-vitro studies, which are conducted outside of living organisms. In-vitro studies, derived from the Latin term "in glass," involve experiments performed in controlled laboratory settings using cells or tissues. It is important to note that these products are not categorized as medicines or drugs, and they have not received approval from the FDA for the prevention, treatment, or cure of any medical condition, ailment, or disease. We must emphasize that any form of bodily introduction of these products into humans or animals is strictly prohibited by law. It is essential to adhere to these guidelines to ensure compliance with legal and ethical standards in research and experimentation.