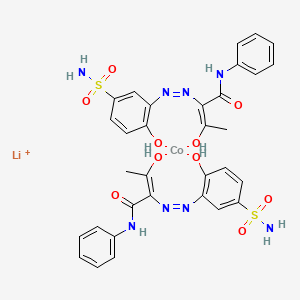
Lithium bis(2-((5-(aminosulphonyl)-2-hydroxyphenyl)azo)-3-oxo-N-phenylbutyramidato(2-))cobaltate(1-)
- Click on QUICK INQUIRY to receive a quote from our team of experts.
- With the quality product at a COMPETITIVE price, you can focus more on your research.
Overview
Description
Lithium bis[2-[[5-(aminosulfonyl)-2-hydroxyphenyl]azo]-3-oxo-N-phenylbutyramidato(2-)]cobaltate(1-) is a coordination compound that features a cobalt center complexed with a ligand containing azo, sulfonamide, and phenyl groups.
Preparation Methods
The synthesis of Lithium bis[2-[[5-(aminosulfonyl)-2-hydroxyphenyl]azo]-3-oxo-N-phenylbutyramidato(2-)]cobaltate(1-) typically involves the reaction of cobalt salts with the appropriate azo ligand under controlled conditions. The ligand is synthesized through a series of steps, starting with the diazotization of 5-aminosulfonyl-2-hydroxyaniline, followed by coupling with 3-oxo-N-phenylbutyramide. The resulting ligand is then reacted with a cobalt salt, such as cobalt(II) chloride, in the presence of lithium ions to form the final complex .
Industrial production methods may involve similar synthetic routes but are optimized for large-scale production. This includes the use of automated reactors, precise control of reaction parameters, and purification techniques to ensure high yield and purity of the final product .
Chemical Reactions Analysis
Lithium bis[2-[[5-(aminosulfonyl)-2-hydroxyphenyl]azo]-3-oxo-N-phenylbutyramidato(2-)]cobaltate(1-) can undergo various chemical reactions, including:
Oxidation and Reduction: The cobalt center can participate in redox reactions, where it can be oxidized or reduced depending on the reaction conditions and reagents used.
Substitution Reactions: The ligand framework allows for substitution reactions, where certain groups can be replaced by other functional groups under appropriate conditions.
Coordination Reactions: The compound can form additional coordination complexes with other metal ions or ligands, leading to the formation of more complex structures.
Common reagents used in these reactions include oxidizing agents like hydrogen peroxide, reducing agents like sodium borohydride, and various nucleophiles and electrophiles for substitution reactions. The major products formed depend on the specific reaction conditions and the nature of the reagents used .
Scientific Research Applications
Lithium bis[2-[[5-(aminosulfonyl)-2-hydroxyphenyl]azo]-3-oxo-N-phenylbutyramidato(2-)]cobaltate(1-) has several scientific research applications:
Chemistry: It is used as a catalyst in various organic reactions, including oxidation and coupling reactions. Its unique structure allows it to facilitate these reactions efficiently.
Biology: The compound’s ability to interact with biological molecules makes it a potential candidate for use in biochemical assays and as a probe for studying enzyme activities.
Medicine: Research is ongoing to explore its potential as a therapeutic agent, particularly in the field of cancer treatment, where its ability to generate reactive oxygen species can be harnessed to target cancer cells.
Industry: It is used in the production of dyes and pigments due to its vibrant color and stability.
Mechanism of Action
The mechanism of action of Lithium bis[2-[[5-(aminosulfonyl)-2-hydroxyphenyl]azo]-3-oxo-N-phenylbutyramidato(2-)]cobaltate(1-) involves its interaction with molecular targets through coordination and redox processes. The cobalt center can undergo redox cycling, generating reactive oxygen species that can induce oxidative stress in biological systems. This property is particularly useful in therapeutic applications, where controlled oxidative stress can be used to target specific cells or tissues .
Comparison with Similar Compounds
Similar compounds include other cobalt-based coordination complexes with azo ligands. Examples include:
- Cobalt bis[2-[[5-(aminosulfonyl)-2-hydroxyphenyl]azo]-3-oxo-N-phenylbutyramidato(2-)]
- Nickel bis[2-[[5-(aminosulfonyl)-2-hydroxyphenyl]azo]-3-oxo-N-phenylbutyramidato(2-)]
- Copper bis[2-[[5-(aminosulfonyl)-2-hydroxyphenyl]azo]-3-oxo-N-phenylbutyramidato(2-)]
Lithium bis[2-[[5-(aminosulfonyl)-2-hydroxyphenyl]azo]-3-oxo-N-phenylbutyramidato(2-)]cobaltate(1-) is unique due to the presence of lithium ions, which can influence its solubility and reactivity. Additionally, the specific arrangement of the ligand around the cobalt center imparts distinct electronic and steric properties, making it suitable for specific applications that other similar compounds may not be able to achieve .
Properties
CAS No. |
83249-68-7 |
---|---|
Molecular Formula |
C32H32CoLiN8O10S2+ |
Molecular Weight |
818.7 g/mol |
IUPAC Name |
lithium;cobalt;(Z)-3-hydroxy-2-[(2-hydroxy-5-sulfamoylphenyl)diazenyl]-N-phenylbut-2-enamide |
InChI |
InChI=1S/2C16H16N4O5S.Co.Li/c2*1-10(21)15(16(23)18-11-5-3-2-4-6-11)20-19-13-9-12(26(17,24)25)7-8-14(13)22;;/h2*2-9,21-22H,1H3,(H,18,23)(H2,17,24,25);;/q;;;+1/b2*15-10-,20-19?;; |
InChI Key |
ZNPLDXZSZZHCSB-QXFYPXFPSA-N |
Isomeric SMILES |
[Li+].C/C(=C(/N=NC1=C(C=CC(=C1)S(=O)(=O)N)O)\C(=O)NC2=CC=CC=C2)/O.C/C(=C(/N=NC1=C(C=CC(=C1)S(=O)(=O)N)O)\C(=O)NC2=CC=CC=C2)/O.[Co] |
Canonical SMILES |
[Li+].CC(=C(C(=O)NC1=CC=CC=C1)N=NC2=C(C=CC(=C2)S(=O)(=O)N)O)O.CC(=C(C(=O)NC1=CC=CC=C1)N=NC2=C(C=CC(=C2)S(=O)(=O)N)O)O.[Co] |
Origin of Product |
United States |
Disclaimer and Information on In-Vitro Research Products
Please be aware that all articles and product information presented on BenchChem are intended solely for informational purposes. The products available for purchase on BenchChem are specifically designed for in-vitro studies, which are conducted outside of living organisms. In-vitro studies, derived from the Latin term "in glass," involve experiments performed in controlled laboratory settings using cells or tissues. It is important to note that these products are not categorized as medicines or drugs, and they have not received approval from the FDA for the prevention, treatment, or cure of any medical condition, ailment, or disease. We must emphasize that any form of bodily introduction of these products into humans or animals is strictly prohibited by law. It is essential to adhere to these guidelines to ensure compliance with legal and ethical standards in research and experimentation.