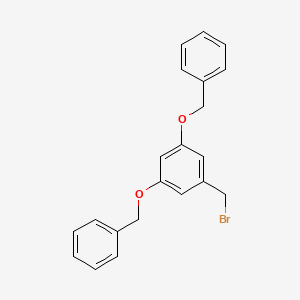
3,5-Dibenzyloxybenzyl Bromide
Overview
Description
“3,5-Dibenzyloxybenzyl Bromide” is a chemical compound with the molecular formula C21H19BrO2 and a molecular weight of 383.29 . It appears as a white to almost white powder or crystal .
Molecular Structure Analysis
The molecular structure of “3,5-Dibenzyloxybenzyl Bromide” consists of a benzyl bromide core with two benzyloxy groups attached at the 3 and 5 positions .
Physical And Chemical Properties Analysis
“3,5-Dibenzyloxybenzyl Bromide” is a solid at 20 degrees Celsius . It has a melting point ranging from 85.0 to 89.0 degrees Celsius . It should be stored at a temperature between 0-10°C and should be kept away from heat .
Scientific Research Applications
Synthesis of Dendrimers
Dendrimers are highly branched, star-shaped macromolecules with potential applications in drug delivery, gene therapy, and as contrast agents in medical imaging3,5-Dibenzyloxybenzyl Bromide serves as a key building block in the synthesis of dendrimers due to its bifunctional nature, allowing for the creation of complex, multi-layered structures .
Safety and Hazard Research
Given its hazardous nature, as indicated by its safety data sheet, research is conducted on the safe handling and disposal of 3,5-Dibenzyloxybenzyl Bromide . This research is crucial for developing protocols to mitigate risks associated with its use in laboratory settings .
Safety and Hazards
Mechanism of Action
Target of Action
It is known that benzylic halides, such as this compound, are particularly reactive . The carbon atom attached to the aromatic ring, known as the benzylic position, is the site of this reactivity .
Mode of Action
3,5-Dibenzyloxybenzyl Bromide, being a benzylic halide, can undergo typical reactions of alkyl halides . The bromine atom in the compound can be replaced by nucleophiles in a nucleophilic substitution reaction . This reactivity at the benzylic position allows the compound to be used frequently in multistep syntheses .
properties
IUPAC Name |
1-(bromomethyl)-3,5-bis(phenylmethoxy)benzene | |
---|---|---|
Source | PubChem | |
URL | https://pubchem.ncbi.nlm.nih.gov | |
Description | Data deposited in or computed by PubChem | |
InChI |
InChI=1S/C21H19BrO2/c22-14-19-11-20(23-15-17-7-3-1-4-8-17)13-21(12-19)24-16-18-9-5-2-6-10-18/h1-13H,14-16H2 | |
Source | PubChem | |
URL | https://pubchem.ncbi.nlm.nih.gov | |
Description | Data deposited in or computed by PubChem | |
InChI Key |
WGMYJGAUAQXYFQ-UHFFFAOYSA-N | |
Source | PubChem | |
URL | https://pubchem.ncbi.nlm.nih.gov | |
Description | Data deposited in or computed by PubChem | |
Canonical SMILES |
C1=CC=C(C=C1)COC2=CC(=CC(=C2)CBr)OCC3=CC=CC=C3 | |
Source | PubChem | |
URL | https://pubchem.ncbi.nlm.nih.gov | |
Description | Data deposited in or computed by PubChem | |
Molecular Formula |
C21H19BrO2 | |
Source | PubChem | |
URL | https://pubchem.ncbi.nlm.nih.gov | |
Description | Data deposited in or computed by PubChem | |
DSSTOX Substance ID |
DTXSID00375482 | |
Record name | 3,5-Dibenzyloxybenzyl Bromide | |
Source | EPA DSSTox | |
URL | https://comptox.epa.gov/dashboard/DTXSID00375482 | |
Description | DSSTox provides a high quality public chemistry resource for supporting improved predictive toxicology. | |
Molecular Weight |
383.3 g/mol | |
Source | PubChem | |
URL | https://pubchem.ncbi.nlm.nih.gov | |
Description | Data deposited in or computed by PubChem | |
Product Name |
3,5-Dibenzyloxybenzyl Bromide | |
CAS RN |
24131-32-6 | |
Record name | 3,5-Dibenzyloxybenzyl Bromide | |
Source | EPA DSSTox | |
URL | https://comptox.epa.gov/dashboard/DTXSID00375482 | |
Description | DSSTox provides a high quality public chemistry resource for supporting improved predictive toxicology. | |
Record name | 3,5-Bis(benzyloxy)benzyl Bromide | |
Source | European Chemicals Agency (ECHA) | |
URL | https://echa.europa.eu/information-on-chemicals | |
Description | The European Chemicals Agency (ECHA) is an agency of the European Union which is the driving force among regulatory authorities in implementing the EU's groundbreaking chemicals legislation for the benefit of human health and the environment as well as for innovation and competitiveness. | |
Explanation | Use of the information, documents and data from the ECHA website is subject to the terms and conditions of this Legal Notice, and subject to other binding limitations provided for under applicable law, the information, documents and data made available on the ECHA website may be reproduced, distributed and/or used, totally or in part, for non-commercial purposes provided that ECHA is acknowledged as the source: "Source: European Chemicals Agency, http://echa.europa.eu/". Such acknowledgement must be included in each copy of the material. ECHA permits and encourages organisations and individuals to create links to the ECHA website under the following cumulative conditions: Links can only be made to webpages that provide a link to the Legal Notice page. | |
Retrosynthesis Analysis
AI-Powered Synthesis Planning: Our tool employs the Template_relevance Pistachio, Template_relevance Bkms_metabolic, Template_relevance Pistachio_ringbreaker, Template_relevance Reaxys, Template_relevance Reaxys_biocatalysis model, leveraging a vast database of chemical reactions to predict feasible synthetic routes.
One-Step Synthesis Focus: Specifically designed for one-step synthesis, it provides concise and direct routes for your target compounds, streamlining the synthesis process.
Accurate Predictions: Utilizing the extensive PISTACHIO, BKMS_METABOLIC, PISTACHIO_RINGBREAKER, REAXYS, REAXYS_BIOCATALYSIS database, our tool offers high-accuracy predictions, reflecting the latest in chemical research and data.
Strategy Settings
Precursor scoring | Relevance Heuristic |
---|---|
Min. plausibility | 0.01 |
Model | Template_relevance |
Template Set | Pistachio/Bkms_metabolic/Pistachio_ringbreaker/Reaxys/Reaxys_biocatalysis |
Top-N result to add to graph | 6 |
Feasible Synthetic Routes
Disclaimer and Information on In-Vitro Research Products
Please be aware that all articles and product information presented on BenchChem are intended solely for informational purposes. The products available for purchase on BenchChem are specifically designed for in-vitro studies, which are conducted outside of living organisms. In-vitro studies, derived from the Latin term "in glass," involve experiments performed in controlled laboratory settings using cells or tissues. It is important to note that these products are not categorized as medicines or drugs, and they have not received approval from the FDA for the prevention, treatment, or cure of any medical condition, ailment, or disease. We must emphasize that any form of bodily introduction of these products into humans or animals is strictly prohibited by law. It is essential to adhere to these guidelines to ensure compliance with legal and ethical standards in research and experimentation.