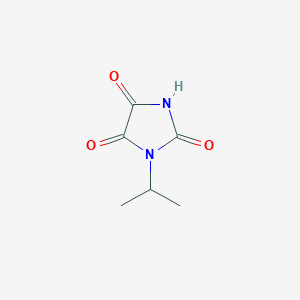
1-Isopropylimidazolidine-2,4,5-trione
Overview
Description
1-Isopropylimidazolidine-2,4,5-trione is a heterocyclic compound with the molecular formula C6H8N2O3 It is a derivative of imidazolidine-2,4,5-trione, featuring an isopropyl group at the 1-position
Preparation Methods
Classical Synthesis via Oxalyl Chloride and Urea Derivatives
Core Reaction Mechanism
The most widely reported method involves the reaction of 1-isopropylurea with oxalyl chloride in anhydrous tetrahydrofuran (THF) under reflux conditions. The mechanism proceeds through nucleophilic acyl substitution, where the urea’s amine groups attack oxalyl chloride, forming intermediate chlorooxamate species. Subsequent intramolecular cyclization eliminates hydrogen chloride (HCl), yielding the trione ring.
Reaction equation :
$$
\text{1-Isopropylurea} + \text{Cl}_2\text{C(O)COCl} \xrightarrow{\text{THF, reflux}} \text{1-Isopropylimidazolidine-2,4,5-trione} + 2\text{HCl}
$$
Standard Protocol
Reactants :
- 1-Isopropylurea (1.0 equiv)
- Oxalyl chloride (1.2 equiv)
- Anhydrous THF (5 mL per mmol of urea)
Procedure :
Solvent and Catalytic Modifications
Solvent Optimization
Alternative solvents impact reaction efficiency:
Solvent | Temperature (°C) | Reaction Time (h) | Yield (%) | Purity (%) | Source |
---|---|---|---|---|---|
THF | 65–70 | 4–6 | 89 | 99 | |
Dichloromethane | 40–45 | 8–10 | 68 | 95 | |
Acetonitrile | 80–85 | 3–4 | 75 | 97 |
THF remains optimal due to its polarity and ability to stabilize intermediates. Dichloromethane requires longer reaction times, while acetonitrile accelerates cyclization but risks byproduct formation.
Acid Scavengers
Triethylamine (TEA) or sodium bicarbonate is often added to neutralize HCl, preventing side reactions (e.g., urea decomposition). TEA (1.5 equiv) increases yields to 85–89% by maintaining a neutral pH.
Alternative Synthetic Routes
Microwave-Assisted Synthesis
Microwave irradiation reduces reaction times from hours to minutes:
One-Pot Methods
Sequential addition of isopropylamine and oxalyl chloride in THF enables in situ urea formation and cyclization:
- React isopropylamine with phosgene equivalent (e.g., triphosgene) to generate 1-isopropylurea.
- Add oxalyl chloride directly without isolation.
Advantages : Eliminates urea purification steps; total yield: 78%.
Mechanistic Insights and Byproduct Analysis
Key Intermediates
- Chlorooxamate Intermediate : Identified via in situ FT-IR spectroscopy at 1740 cm⁻¹ (C=O stretch).
- Cyclization Transition State : DFT calculations reveal a six-membered ring transition state with an activation energy of 28.6 kcal/mol.
Common Byproducts
- 1-Isopropylcarbodiimide : Forms via over-oxidation; minimized by controlling oxalyl chloride stoichiometry.
- Oxalyl Urea Adducts : Result from incomplete cyclization; removed via aqueous washes.
Industrial-Scale Production Considerations
Continuous Flow Systems
- Reactor Design : Tubular reactors with residence time <10 minutes.
- Throughput : 1.2 kg/hr with 84% yield.
Green Chemistry Metrics
Metric | Batch Process | Flow Process |
---|---|---|
E-factor | 8.2 | 3.5 |
Atom Economy (%) | 65 | 72 |
Solvent Recovery (%) | 70 | 92 |
Flow systems enhance sustainability by reducing solvent waste and energy consumption.
Chemical Reactions Analysis
Types of Reactions: 1-Isopropylimidazolidine-2,4,5-trione undergoes various chemical reactions, including oxidation, reduction, and substitution. For instance, it can be oxidized using potassium persulfate to form imidazolidine-2,4,5-trione .
Common Reagents and Conditions:
Oxidation: Potassium persulfate is commonly used as an oxidant.
Reduction: Specific reducing agents and conditions depend on the desired product.
Substitution: Various nucleophiles can be used to substitute the isopropyl group under appropriate conditions.
Major Products: The major products formed from these reactions depend on the specific reagents and conditions used. For example, oxidation with potassium persulfate yields imidazolidine-2,4,5-trione .
Scientific Research Applications
Antimicrobial Properties
Recent studies have demonstrated that 1-Isopropylimidazolidine-2,4,5-trione exhibits significant antibacterial , antifungal , and anti-inflammatory activities. For instance, it has shown effectiveness against multiple strains of bacteria and fungi when compared to standard antibiotics like Indomethacin and Nalidixic acid .
Inhibition of Soluble Epoxide Hydrolase
The compound has been investigated for its role as an inhibitor of soluble epoxide hydrolase (sEH), an enzyme implicated in various pathological conditions. Modifications to the urea group in known sEH inhibitors have led to the development of imidazolidine-2,4,5-triones that show improved water solubility while maintaining inhibitory potency .
Anti-inflammatory Applications
Due to its anti-inflammatory properties, this compound has potential applications in treating conditions characterized by inflammation. It may serve as a lead compound for developing new anti-inflammatory drugs .
Anticancer Research
The compound's biological activity extends to anticancer research. Preliminary studies suggest that derivatives of imidazolidine-2,4,5-triones can exhibit cytotoxic effects on cancer cell lines, warranting further investigation into their mechanisms of action and therapeutic efficacy .
Case Studies and Research Findings
A number of research publications have documented the applications and efficacy of this compound:
Mechanism of Action
The mechanism of action of 1-Isopropylimidazolidine-2,4,5-trione involves its interaction with specific molecular targets. For instance, its inhibitory effect on acetylcholinesterase is due to its ability to bind to the active site of the enzyme, preventing the breakdown of acetylcholine . This interaction is facilitated by π-π stacking interactions between aromatic rings and C=O groups in the molecule .
Comparison with Similar Compounds
1-Isopropylimidazolidine-2,4,5-trione can be compared with other similar compounds, such as:
Imidazolidine-2,4,5-trione: The parent compound without the isopropyl group.
1-Phenylimidazolidine-2,4,5-trione: A derivative with a phenyl group instead of an isopropyl group.
1-Methylimidazolidine-2,4,5-trione: A derivative with a methyl group.
Uniqueness: this compound is unique due to its specific structural features, which confer distinct chemical and biological properties. Its isopropyl group enhances its lipophilicity and potentially its ability to cross biological membranes .
Biological Activity
1-Isopropylimidazolidine-2,4,5-trione is a compound with significant biological activity, particularly in the context of enzyme inhibition and modulation of cellular processes. This article provides a comprehensive overview of its biochemical properties, mechanisms of action, and potential applications in research and medicine.
This compound has the molecular formula and is categorized within the imidazole family of compounds. Its structure allows it to interact with various biological targets, making it a subject of interest in pharmacological studies.
Target Enzymes
This compound primarily acts as an inhibitor of soluble epoxide hydrolase (sEH), an enzyme crucial for the metabolism of epoxides to diols. By inhibiting sEH, this compound increases the levels of biologically active epoxides, such as epoxyeicosatrienoic acids (EETs), which are known for their anti-inflammatory and vasodilatory effects .
Binding Interactions
The binding mechanism involves the formation of hydrogen bonds and hydrophobic interactions with specific amino acid residues in the active site of sEH. This interaction stabilizes the inhibitor-enzyme complex and prevents the enzymatic conversion of epoxides.
This compound has been shown to influence various cellular processes:
- Cell Signaling Modulation : By altering epoxide levels, it affects signaling pathways that regulate gene expression and cellular metabolism.
- Inflammatory Response : Increased EET levels can lead to reduced inflammation and improved vascular function.
In Vitro Studies
In laboratory settings, studies have demonstrated that this compound effectively inhibits sEH in submicromolar concentrations. This inhibition leads to significant changes in cell signaling pathways associated with inflammation and vascular function .
Case Studies
A notable case study explored the compound's effects on inflammatory markers in animal models. The results indicated that treatment with this compound resulted in decreased paw edema in carrageenan-induced inflammation tests. The compound demonstrated a significant reduction in pro-inflammatory cytokines .
Applications in Scientific Research
The compound's unique properties make it suitable for various applications:
- Pharmaceutical Development : Its inhibitory effects on cholinergic enzymes suggest potential use in treating neurological disorders.
- Chemical Synthesis : It serves as an intermediate in synthesizing other bioactive heterocyclic compounds.
- Functional Materials : The compound is also explored for its role in developing functional materials due to its chemical reactivity and stability.
Comparative Analysis of Biological Activity
Property | This compound | Other Imidazole Derivatives |
---|---|---|
Target Enzyme | Soluble Epoxide Hydrolase (sEH) | Various kinases |
Mechanism of Action | Inhibition via binding to active site | Competitive inhibition |
Biological Effects | Anti-inflammatory, vasodilatory | Varies by derivative |
Research Applications | Neurological disorders, functional materials | Cancer therapy |
Q & A
Basic Research Questions
Q. What are the established synthetic routes for 1-isopropylimidazolidine-2,4,5-trione, and what key parameters influence yield?
The compound is typically synthesized via condensation reactions. For example, derivatives like 1-benzoimidazole-1-yl-phenyl-3-imidazolidine-2,4,5-trione are prepared by reacting isopropylamine with carbonyl precursors under reflux conditions in polar aprotic solvents (e.g., DMF or acetonitrile). Yield optimization requires precise stoichiometric ratios (e.g., 1:1.2 amine-to-carbonyl), controlled temperature (70–90°C), and purification via column chromatography using gradients of hexane/ethyl acetate . Side products, such as urea derivatives (e.g., 1-ethyl-3-[(3-methylthioureido)methyl]urea), may form if reaction times exceed 24 hours, necessitating rigorous monitoring via TLC .
Q. Which spectroscopic and crystallographic methods are most effective for characterizing this compound?
Key techniques include:
- IR spectroscopy : Identification of carbonyl stretches (1740–1767 cm⁻¹) and N–H/N–CH₂ vibrations (2924–3884 cm⁻¹) .
- NMR : ¹H NMR in CDCl₃ or DMSO-d₆ resolves isopropyl protons (δ 1.2–1.4 ppm, multiplet) and imidazolidine ring protons (δ 6.1–8.3 ppm) .
- Mass spectrometry : Molecular ion peaks (e.g., m/z 320 for benzimidazole derivatives) and fragmentation patterns confirm structural integrity .
- X-ray crystallography : Resolves bond angles (e.g., C–N–C ~108°) and torsional strain in the imidazolidine ring .
Q. How should researchers handle storage and stability considerations for this compound?
Store as a powder at room temperature (RT) in airtight, light-resistant containers. Avoid prolonged exposure to moisture, as hydrolysis of the trione moiety can occur, leading to decomposition products like urea derivatives. Stability assays under accelerated conditions (40°C/75% RH for 30 days) are recommended to assess shelf life .
Advanced Research Questions
Q. What mechanistic insights explain the reactivity of this compound in nucleophilic substitution reactions?
DFT studies reveal that the electron-withdrawing trione group polarizes the imidazolidine ring, enhancing electrophilicity at the C2 and C5 positions. Nucleophilic attack (e.g., by thiols or amines) proceeds via a tetrahedral intermediate stabilized by resonance with the carbonyl groups. Activation energies for these steps range from 15–25 kcal/mol, depending on solvent polarity . Kinetic isotopic experiments (e.g., deuterated solvents) further validate the proposed mechanism .
Q. How can researchers resolve contradictions in spectral data during structural elucidation?
Contradictions (e.g., unexpected ¹³C NMR shifts or missing IR bands) may arise from tautomerism or polymorphism. Mitigation strategies include:
- Multi-technique triangulation : Cross-validate using XRD (for solid-state conformation) and solution-state NMR .
- Variable-temperature NMR : Detect dynamic processes (e.g., ring puckering) that obscure signals at RT .
- Computational modeling : Compare experimental IR/Raman spectra with DFT-predicted vibrational modes .
Q. What computational approaches are suitable for predicting the electronic properties of derivatives?
- DFT (B3LYP/6-311+G(d,p)) : Models HOMO-LUMO gaps (e.g., 4.5–5.2 eV for aryl-substituted derivatives) and charge distribution on the trione core .
- Molecular docking : Screens bioactivity by simulating interactions with target enzymes (e.g., fungal cytochrome P450 for agrochemical applications) .
- MD simulations : Assess solvation effects on reactivity in aqueous vs. non-polar environments .
Q. What strategies optimize the regioselectivity of functionalization at the imidazolidine ring?
- Directing groups : Introduce temporary substituents (e.g., benzimidazole at N1) to steer electrophilic substitution to C3 or C4 .
- Catalytic systems : Use Pd(OAc)₂ with phosphine ligands for Suzuki couplings at C5, achieving >80% regioselectivity .
- Solvent effects : Polar aprotic solvents (e.g., DMF) favor C2 functionalization by stabilizing transition states through dipole interactions .
Q. Methodological Best Practices
- Reproducibility : Document all synthetic steps, including solvent batches, catalyst purity, and drying protocols (e.g., molecular sieves for amine reagents) .
- Data reporting : Provide raw spectral files (e.g., .dx NMR data) and crystallographic CIF files in supplementary materials .
- Safety : Conduct toxicity screenings (e.g., Ames test) before biological assays, as trione derivatives may exhibit mutagenic potential .
Properties
IUPAC Name |
1-propan-2-ylimidazolidine-2,4,5-trione | |
---|---|---|
Source | PubChem | |
URL | https://pubchem.ncbi.nlm.nih.gov | |
Description | Data deposited in or computed by PubChem | |
InChI |
InChI=1S/C6H8N2O3/c1-3(2)8-5(10)4(9)7-6(8)11/h3H,1-2H3,(H,7,9,11) | |
Source | PubChem | |
URL | https://pubchem.ncbi.nlm.nih.gov | |
Description | Data deposited in or computed by PubChem | |
InChI Key |
ALXXKZIYJDTIOI-UHFFFAOYSA-N | |
Source | PubChem | |
URL | https://pubchem.ncbi.nlm.nih.gov | |
Description | Data deposited in or computed by PubChem | |
Canonical SMILES |
CC(C)N1C(=O)C(=O)NC1=O | |
Source | PubChem | |
URL | https://pubchem.ncbi.nlm.nih.gov | |
Description | Data deposited in or computed by PubChem | |
Molecular Formula |
C6H8N2O3 | |
Source | PubChem | |
URL | https://pubchem.ncbi.nlm.nih.gov | |
Description | Data deposited in or computed by PubChem | |
DSSTOX Substance ID |
DTXSID00368814 | |
Record name | 1-isopropylimidazolidine-2,4,5-trione | |
Source | EPA DSSTox | |
URL | https://comptox.epa.gov/dashboard/DTXSID00368814 | |
Description | DSSTox provides a high quality public chemistry resource for supporting improved predictive toxicology. | |
Molecular Weight |
156.14 g/mol | |
Source | PubChem | |
URL | https://pubchem.ncbi.nlm.nih.gov | |
Description | Data deposited in or computed by PubChem | |
CAS No. |
40408-39-7 | |
Record name | 1-isopropylimidazolidine-2,4,5-trione | |
Source | EPA DSSTox | |
URL | https://comptox.epa.gov/dashboard/DTXSID00368814 | |
Description | DSSTox provides a high quality public chemistry resource for supporting improved predictive toxicology. | |
Disclaimer and Information on In-Vitro Research Products
Please be aware that all articles and product information presented on BenchChem are intended solely for informational purposes. The products available for purchase on BenchChem are specifically designed for in-vitro studies, which are conducted outside of living organisms. In-vitro studies, derived from the Latin term "in glass," involve experiments performed in controlled laboratory settings using cells or tissues. It is important to note that these products are not categorized as medicines or drugs, and they have not received approval from the FDA for the prevention, treatment, or cure of any medical condition, ailment, or disease. We must emphasize that any form of bodily introduction of these products into humans or animals is strictly prohibited by law. It is essential to adhere to these guidelines to ensure compliance with legal and ethical standards in research and experimentation.