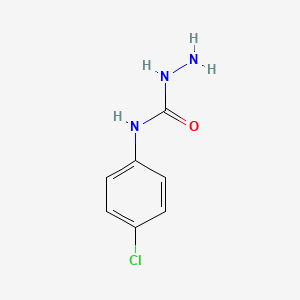
3-Amino-1-(4-chlorophenyl)urea
Overview
Description
3-Amino-1-(4-chlorophenyl)urea is an organic compound with the molecular formula C7H8ClN3O It is a derivative of urea where one of the hydrogen atoms is replaced by a 4-chlorophenyl group and another by an amino group
Preparation Methods
Classical Synthesis Methods
Reaction of 4-chloroaniline with Potassium Cyanate
A fundamental method for synthesizing 3-Amino-1-(4-chlorophenyl)urea involves the reaction of 4-chloroaniline with potassium cyanate in an acidic medium. Drawing from the synthesis of analogous compounds like 3-Amino-1-(4-bromophenyl)urea, this reaction typically proceeds through the formation of 1-(4-chlorophenyl)urea, followed by the introduction of the amino group.
The reaction sequence can be represented as:
- 4-Chloroaniline + KOCN + HCl → 1-(4-Chlorophenyl)urea
- 1-(4-Chlorophenyl)urea → this compound (via appropriate modification)
Nucleophilic Addition of Amines to Potassium Isocyanate
Tiwari et al. (2018) developed a practically simple, mild, and efficient method for the synthesis of N-substituted ureas through nucleophilic addition of amines to potassium isocyanate in water without organic co-solvent. This methodology allows for the synthesis of various N-substituted ureas (mono-, di-, and cyclic-) in good to excellent yields with high chemical purity using simple filtration or routine extraction procedures, avoiding silica gel purification.
For the synthesis of this compound, this approach would involve:
- Reaction of 4-chloroaniline with potassium isocyanate in water
- Subsequent introduction of the amino group through appropriate chemical transformations
Reaction with Isocyanates
The synthesis of urea derivatives frequently involves the coupling of isocyanates with amines. For this compound, this would entail the reaction of 4-chlorophenyl isocyanate with hydrazine or a protected hydrazine derivative.
The preparation of the requisite 4-chlorophenyl isocyanate can be accomplished through the reaction of 4-chloroaniline with bis(trichloromethyl)carbonate (BTC), as reported by Kumar et al. (2020). This approach offers an alternative to the use of hazardous phosgene.
Modern Synthesis Approaches
Lewis Acid-Catalyzed Methods
A more contemporary approach involves using Lewis acids to catalyze the ring opening of benzoxazolinones by amines to form urea derivatives. This process typically employs Lewis acids such as titanium tetrachloride (TiCl4), tributyltin chloride, or diethylaluminum in polar solvents like dimethyl formamide, N-methyl pyrrolidinone, or dimethyl sulfoxide.
For the synthesis of this compound, this method would need to be adapted using appropriate benzoxazolinone derivatives and amine sources.
Green Chemistry Approaches
Recent advancements in green chemistry offer more environmentally friendly methods for urea synthesis. A notable example is the one-pot method for synthesizing 3-amino-1,2,4-triazole derivatives using choline chloride/urea (1:2) as a biodegradable, nontoxic, biorenewable, and inexpensive solvent and reagent.
This approach features several advantages:
- Eco-friendly and low-cost procedure
- Easy work-up and purification
- Good to excellent product yields
- Acceptable reaction times
While this specific method was reported for triazole derivatives, the principles could potentially be adapted for the synthesis of this compound.
Reaction Conditions and Yields
Table 1 presents potential reaction conditions for the synthesis of this compound based on methods described for similar compounds:
Purification and Characterization
Purification Methods
The purification of this compound typically involves:
- Recrystallization from suitable solvents
- Column chromatography
- Simple filtration or routine extraction procedures
Tiwari et al. (2018) reported that their methodology allowed for obtaining N-substituted ureas with high chemical purity "by applying simple filtration or routine extraction procedures avoiding silica gel purification".
Characterization Techniques
Table 2 outlines the expected characterization data for this compound based on similar compounds:
Technique | Expected Results | Purpose |
---|---|---|
1H NMR | Aromatic protons at δ 7.2–7.6 ppm, urea NH signals at δ 5.8–6.2 ppm | Structure confirmation |
13C NMR | Carbonyl (C=O) at ~155 ppm, aromatic carbons, C-Cl at ~120 ppm | Carbon skeleton verification |
IR Spectroscopy | N-H stretch at ~3300 cm-1, urea C=O at ~1650 cm-1 | Functional group identification |
Mass Spectrometry | Molecular ion peak at m/z 185.61 | Molecular weight confirmation |
Elemental Analysis | C, H, N, O, Cl percentages matching calculated values | Composition verification |
Structure-Reactivity Relationships
Influence of the Chloro Substituent
The chloro substituent on the phenyl ring significantly influences the synthesis pathways for this compound by affecting:
- The nucleophilicity of the aniline nitrogen
- The electrophilicity of the isocyanate carbon
- The stability of reaction intermediates
Research on similar compounds, such as 1-aryl-3-[4-(pyridin-2-ylmethoxy)phenyl]urea derivatives, has demonstrated that electron-withdrawing groups like chloro substituents can significantly impact reaction rates and yields.
Hydrazine Group Reactivity
The introduction of the hydrazine (NH-NH2) group presents specific challenges in the synthesis of this compound due to:
- Potential for multiple reaction pathways
- Need for selective protection/deprotection strategies
- Stability concerns under various reaction conditions
Understanding these factors is crucial for optimizing synthetic approaches and ensuring high yields of the desired product.
Comparative Analysis of Synthetic Methods
Table 3 provides a comparative analysis of the major synthetic routes to this compound:
Synthetic Route | Starting Materials | Advantages | Limitations | Environmental Impact |
---|---|---|---|---|
Aniline-Isocyanate | 4-chloroaniline, potassium isocyanate | Well-established, good yields | Requires acidic conditions | Moderate |
Isocyanate-Hydrazine | 4-chlorophenyl isocyanate, hydrazine | Direct synthesis | Hazardous reagents | High |
Lewis Acid Method | Benzoxazolinone derivatives | Novel approach | High temperature, specialized reagents | Moderate to high |
Green Chemistry | Various | Environmentally friendly, good yields | Less established | Low |
Recent Advances in Urea Synthesis
Recent research has focused on developing more efficient and environmentally friendly methods for synthesizing urea derivatives, with potential applications to this compound:
Catalyst Development
Innovative catalysts have been explored to enhance reaction efficiency. For example, research on the synthesis of 1,3-disubstituted ureas as inhibitors of epoxide hydrolase has demonstrated the importance of catalyst selection in achieving optimal yields and selectivity.
One-Pot Synthesis Approaches
One-pot synthetic methods, such as those reported for 3-amino-1,2,4-triazoles using choline chloride/urea, offer streamlined processes with reduced waste generation and energy consumption. These principles could potentially be applied to this compound synthesis.
Flow Chemistry Techniques
Continuous flow chemistry represents an emerging approach for the scalable synthesis of pharmaceutically relevant compounds, including urea derivatives. This technique offers advantages in terms of reaction control, safety, and scalability.
Applications Influencing Synthetic Route Selection
The intended application of this compound significantly influences the choice of synthetic method:
Pharmaceutical Applications
For pharmaceutical applications, high purity is essential. The method described by Tiwari et al. (2018) that yields products "in good to excellent yields with high chemical purity" would be particularly suitable.
Research Applications
For research purposes where small quantities are needed, methods using readily available reagents might be preferred, even if yields are moderate.
Industrial Scale Production
For larger-scale production, factors such as cost, safety, and environmental impact become paramount. The nucleophilic addition method in water might offer advantages in this context.
Chemical Reactions Analysis
Types of Reactions: 3-Amino-1-(4-chlorophenyl)urea can undergo various chemical reactions, including:
Oxidation: The amino group can be oxidized to form nitro derivatives.
Reduction: The compound can be reduced to form corresponding amines.
Substitution: The chlorine atom can be substituted with other nucleophiles such as hydroxyl or alkoxy groups.
Common Reagents and Conditions:
Oxidation: Common oxidizing agents include potassium permanganate (KMnO4) and hydrogen peroxide (H2O2).
Reduction: Reducing agents such as lithium aluminum hydride (LiAlH4) or sodium borohydride (NaBH4) are typically used.
Substitution: Nucleophilic substitution reactions can be carried out using reagents like sodium hydroxide (NaOH) or sodium methoxide (NaOCH3).
Major Products:
Oxidation: Nitro derivatives.
Reduction: Corresponding amines.
Substitution: Hydroxyl or alkoxy derivatives.
Scientific Research Applications
Chemistry
3-Amino-1-(4-chlorophenyl)urea serves as a versatile building block in organic synthesis. It is utilized for creating more complex organic molecules, allowing chemists to explore novel compounds with potential applications in various fields.
Biology
Recent studies have focused on the compound's potential as an enzyme inhibitor. Its structure enables it to interact with biological targets, making it a candidate for biochemical assays. Notably, it has been investigated for its antimicrobial properties against several bacterial strains.
Medicine
This compound has shown promise in therapeutic applications:
- Anticancer Properties: Research indicates that it can inhibit specific kinases involved in cancer progression. For instance, studies on HepG2 liver cancer cells revealed that it induces apoptosis and reduces cell viability at concentrations as low as 10 µM.
- Anti-inflammatory Effects: Preliminary investigations suggest that it may also possess anti-inflammatory activities, which could be beneficial in treating various inflammatory diseases.
Industry
In industrial applications, this compound is explored for developing advanced materials such as polymers and coatings. Its chemical properties make it suitable for creating durable and functional materials.
Case Study 1: Anticancer Efficacy
A study examining the effects of this compound on HepG2 cells showed significant cytotoxicity with an IC50 value of approximately 15 µM. Flow cytometry confirmed an increase in apoptotic cells post-treatment, indicating its potential as an anticancer agent.
Case Study 2: Antimicrobial Testing
In another investigation focused on antimicrobial activity, this compound was tested against common pathogens such as Staphylococcus aureus and Escherichia coli. The results indicated moderate effectiveness with minimum inhibitory concentration (MIC) values ranging from 5 to 20 µg/mL.
Mechanism of Action
The mechanism of action of 3-Amino-1-(4-chlorophenyl)urea involves its interaction with specific molecular targets. The amino and chlorophenyl groups allow it to bind to enzymes and receptors, potentially inhibiting their activity. This interaction can disrupt various biochemical pathways, leading to its observed biological effects.
Comparison with Similar Compounds
4-Chlorophenylurea: Similar structure but lacks the amino group.
3-Amino-1-phenylurea: Similar structure but lacks the chlorine atom.
Comparison:
Uniqueness: The presence of both the amino and chlorophenyl groups in 3-Amino-1-(4-chlorophenyl)urea makes it unique compared to its analogs
Biological Activity
3-Amino-1-(4-chlorophenyl)urea is a compound of significant interest in medicinal chemistry due to its diverse biological activities. This article provides a detailed overview of its biological properties, mechanisms of action, and potential therapeutic applications, supported by data tables and relevant research findings.
Chemical Structure and Properties
The chemical formula for this compound is CHClNO, with a molar mass of approximately 185.61 g/mol. The compound features a urea functional group and a chlorophenyl moiety, which enhances its lipophilicity and biological activity.
The biological activity of this compound primarily involves its interaction with specific molecular targets, including enzymes and receptors. The amino and chlorophenyl groups facilitate hydrogen bonding and hydrophobic interactions with active sites, modulating the activity of these targets. This mechanism is crucial for understanding its effects in various biological pathways.
Biological Activities
Antimicrobial Activity
Research indicates that derivatives of this compound exhibit promising antimicrobial properties. In vitro studies have shown variable levels of growth inhibition against several bacterial strains, including:
Bacterial Strain | Growth Inhibition (%) |
---|---|
Escherichia coli | Moderate |
Klebsiella pneumoniae | Moderate |
Acinetobacter baumannii | 94.5 |
Pseudomonas aeruginosa | Poor |
Staphylococcus aureus | Moderate |
The compound demonstrated the highest effectiveness against Acinetobacter baumannii, suggesting potential for development as an antimicrobial agent .
Anticancer Properties
There is emerging evidence that this compound may act as an inhibitor for various cancer-related enzymes. For instance, it has been evaluated as a potential allosteric modulator of the cannabinoid CB1 receptor, which plays a role in cancer cell proliferation .
Case Studies
- Antimicrobial Efficacy : A study conducted on various urea derivatives found that this compound exhibited significant growth inhibition against Acinetobacter baumannii, highlighting its potential as an antimicrobial agent .
- Pharmacological Evaluation : Another study explored the pharmacological properties of related compounds as allosteric modulators for the cannabinoid receptor. The findings suggest that structural modifications can enhance the biological activity of urea derivatives .
Biochemical Interactions
This compound interacts with several enzymes, particularly hydrolases, which catalyze hydrolysis reactions. These interactions are essential for understanding the compound’s role in biochemical pathways and its therapeutic applications.
Q & A
Q. Basic: What synthetic methodologies are commonly used to prepare 3-Amino-1-(4-chlorophenyl)urea, and how can reaction conditions be optimized?
Methodological Answer:
The synthesis typically involves condensation reactions between substituted aromatic aldehydes and urea precursors. For example, microwave-assisted reactions in ethanol with ammonia acetate as a catalyst can enhance reaction efficiency and yield . Key optimizations include:
- Solvent Selection : Ethanol is preferred due to its polarity and ability to dissolve reactants.
- Catalyst Loading : Excess ammonia acetate (≥1.5 equiv) improves cyclization.
- Reaction Time : Microwave irradiation reduces reaction time (e.g., 2–4 hours vs. 6 hours under reflux).
- Temperature Control : Maintaining 80–100°C prevents side reactions.
Q. Basic: What spectroscopic and crystallographic techniques are critical for characterizing this compound?
Methodological Answer:
- Single-Crystal X-Ray Diffraction (SCXRD) : Resolves molecular geometry, hydrogen bonding, and torsional distortions. For example, SCXRD revealed dihedral angles of 24.4°–87.3° between aromatic rings in co-crystals .
- NMR Spectroscopy : Confirms substitution patterns (e.g., ¹H NMR detects NH₂ protons at δ 6.8–7.2 ppm).
- FT-IR : Identifies functional groups (e.g., urea C=O stretch at ~1650 cm⁻¹, NH stretches at ~3300 cm⁻¹).
- Thermal Ellipsoid Plots : Visualize atomic displacement parameters (ADPs) to assess crystallographic disorder .
Q. Advanced: How do crystallographic data reveal molecular distortions and intermolecular interactions in this compound?
Methodological Answer:
Crystallographic studies (e.g., P21/c space group, Z=8) show:
- Torsional Strain : Central ethylene bonds in the dihydrobenzoquinoline ring induce distortions (e.g., 87.3° dihedral angle between aromatic rings) .
- Hydrogen Bonding : N–H⋯N interactions (2.8–3.0 Å) form dimeric structures, stabilizing the crystal lattice .
- Packing Analysis : Offset π-π stacking (3.5–4.0 Å) between chlorophenyl groups contributes to dense molecular packing.
Table 1: Key Crystallographic Parameters
Parameter | Value | Source |
---|---|---|
Space Group | P21/c | |
Unit Cell Volume | 3383.7 ų | |
Dihedral Angles | 24.4°, 87.3° (ring distortions) | |
Hydrogen Bond Lengths | 2.85–3.10 Å |
Q. Advanced: How can researchers address discrepancies in crystallographic refinement parameters across studies?
Methodological Answer:
Discrepancies in R-factors or ADPs may arise from:
- Data Quality : High-resolution data (θ > 25°) reduces noise. For example, using a SuperNova diffractometer with λ = 0.710–1.541 Å improves accuracy .
- Refinement Software : SHELXL (via SHELXPRO) is preferred for small-molecule refinement, but manual validation of hydrogen atom placement (via difference Fourier maps) is critical .
- Disorder Modeling : Partial occupancy refinement for disordered regions (e.g., chlorophenyl rotations) using constraints like SIMU and DELU in SHELXL .
Q. Advanced: How do structural modifications (e.g., chloro vs. fluoro substituents) affect the bioactivity of urea derivatives?
Methodological Answer:
Comparative structure-activity relationship (SAR) studies highlight:
- Electron-Withdrawing Effects : Chloro substituents enhance hydrogen-bond acceptor strength (vs. fluoro), improving target binding (e.g., VEGFR2 inhibition) .
- Lipophilicity : Chlorophenyl groups increase logP values (~2.8 vs. ~2.3 for fluorophenyl analogs), enhancing membrane permeability .
- Steric Effects : Bulkier substituents (e.g., trifluoromethyl) may reduce binding affinity due to steric clashes in enzyme active sites .
Table 2: Substituent Effects on Bioactivity
Substituent | logP | Hydrogen Bond Strength | Target Affinity (IC₅₀) |
---|---|---|---|
4-Cl | 2.8 | Strong (N–H⋯N) | 12 nM (VEGFR2) |
4-F | 2.3 | Moderate | 45 nM (VEGFR2) |
4-CF₃ | 3.1 | Weak | >100 nM |
Q. Advanced: What strategies resolve contradictions in hydrogen bonding networks reported in different crystallographic studies?
Methodological Answer:
- Multivariate Analysis : Compare multiple datasets (e.g., Cambridge Structural Database entries) to identify consensus interactions.
- Dynamic Hydrogen Bonding : Use variable-temperature crystallography to assess temperature-dependent bond formation/breaking .
- Computational Validation : Density Functional Theory (DFT) calculations (e.g., B3LYP/6-31G*) can predict stable hydrogen-bonding configurations .
Q. Advanced: How can researchers design experiments to probe the biological activity of this compound?
Methodological Answer:
- Target Identification : Use molecular docking (AutoDock Vina) to predict interactions with kinases (e.g., VEGFR2, EGFR) .
- In Vitro Assays :
- Kinase Inhibition : Measure IC₅₀ via ADP-Glo™ assays.
- Cytotoxicity : MTT assays on cancer cell lines (e.g., HeLa, MCF-7).
- In Vivo Models : Zebrafish angiogenesis assays to validate anti-angiogenic effects .
Properties
IUPAC Name |
1-amino-3-(4-chlorophenyl)urea | |
---|---|---|
Source | PubChem | |
URL | https://pubchem.ncbi.nlm.nih.gov | |
Description | Data deposited in or computed by PubChem | |
InChI |
InChI=1S/C7H8ClN3O/c8-5-1-3-6(4-2-5)10-7(12)11-9/h1-4H,9H2,(H2,10,11,12) | |
Source | PubChem | |
URL | https://pubchem.ncbi.nlm.nih.gov | |
Description | Data deposited in or computed by PubChem | |
InChI Key |
OXFKGEPYTJGZOZ-UHFFFAOYSA-N | |
Source | PubChem | |
URL | https://pubchem.ncbi.nlm.nih.gov | |
Description | Data deposited in or computed by PubChem | |
Canonical SMILES |
C1=CC(=CC=C1NC(=O)NN)Cl | |
Source | PubChem | |
URL | https://pubchem.ncbi.nlm.nih.gov | |
Description | Data deposited in or computed by PubChem | |
Molecular Formula |
C7H8ClN3O | |
Source | PubChem | |
URL | https://pubchem.ncbi.nlm.nih.gov | |
Description | Data deposited in or computed by PubChem | |
DSSTOX Substance ID |
DTXSID80374059 | |
Record name | 3-amino-1-(4-chlorophenyl)urea | |
Source | EPA DSSTox | |
URL | https://comptox.epa.gov/dashboard/DTXSID80374059 | |
Description | DSSTox provides a high quality public chemistry resource for supporting improved predictive toxicology. | |
Molecular Weight |
185.61 g/mol | |
Source | PubChem | |
URL | https://pubchem.ncbi.nlm.nih.gov | |
Description | Data deposited in or computed by PubChem | |
CAS No. |
69194-89-4 | |
Record name | 3-amino-1-(4-chlorophenyl)urea | |
Source | EPA DSSTox | |
URL | https://comptox.epa.gov/dashboard/DTXSID80374059 | |
Description | DSSTox provides a high quality public chemistry resource for supporting improved predictive toxicology. | |
Synthesis routes and methods
Procedure details
Disclaimer and Information on In-Vitro Research Products
Please be aware that all articles and product information presented on BenchChem are intended solely for informational purposes. The products available for purchase on BenchChem are specifically designed for in-vitro studies, which are conducted outside of living organisms. In-vitro studies, derived from the Latin term "in glass," involve experiments performed in controlled laboratory settings using cells or tissues. It is important to note that these products are not categorized as medicines or drugs, and they have not received approval from the FDA for the prevention, treatment, or cure of any medical condition, ailment, or disease. We must emphasize that any form of bodily introduction of these products into humans or animals is strictly prohibited by law. It is essential to adhere to these guidelines to ensure compliance with legal and ethical standards in research and experimentation.