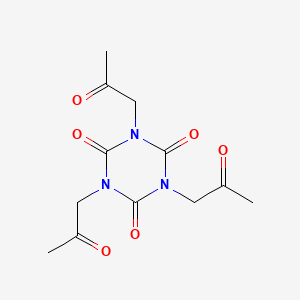
Tris(2-oxopropyl)isocyanurate
- Click on QUICK INQUIRY to receive a quote from our team of experts.
- With the quality product at a COMPETITIVE price, you can focus more on your research.
Overview
Description
Tris(2,3-epoxypropyl)isocyanurate (TGIC), also known as triglycidyl isocyanurate (CAS 2451-62-9), is a trifunctional epoxy compound with the molecular formula C₁₂H₁₅N₃O₆ and a molecular weight of 297.26 g/mol . Its structure consists of a triazine core substituted with three 2,3-epoxypropyl groups, enabling high reactivity in crosslinking applications. TGIC is widely utilized in coatings, adhesives, and UV-curable resins due to its thermal stability and ability to form robust polymer networks . For example, it acts as a crosslinking agent in thiol-ene systems, where UV curing enhances mechanical properties .
TGIC is classified under multiple hazard categories, including acute toxicity (oral/inhalation), skin/eye irritation, and genetic toxicity, necessitating stringent handling protocols .
Preparation Methods
Synthetic Routes and Reaction Conditions: Tris(2-oxopropyl)isocyanurate can be synthesized through a multi-step process involving the reaction of cyanuric acid with propylene oxide. The reaction typically requires a catalyst, such as a base, to facilitate the formation of the oxopropyl groups. The reaction conditions often include moderate temperatures and controlled pH levels to ensure the desired product is obtained .
Industrial Production Methods: In industrial settings, the production of this compound involves large-scale reactors where the reactants are mixed and heated under controlled conditions. The process may also include purification steps to remove any impurities and ensure the final product meets the required specifications .
Chemical Reactions Analysis
Types of Reactions: Tris(2-oxopropyl)isocyanurate undergoes various chemical reactions, including:
Oxidation: This compound can be oxidized to form different products depending on the oxidizing agent used.
Reduction: It can be reduced under specific conditions to yield different derivatives.
Substitution: The oxopropyl groups can be substituted with other functional groups through nucleophilic substitution reactions
Common Reagents and Conditions:
Oxidation: Common oxidizing agents include hydrogen peroxide and potassium permanganate.
Reduction: Reducing agents such as lithium aluminum hydride can be used.
Substitution: Nucleophiles like amines or alcohols are often used in substitution reactions
Major Products Formed: The major products formed from these reactions depend on the specific reagents and conditions used. For example, oxidation may yield carboxylic acids, while reduction can produce alcohols .
Scientific Research Applications
Structural Overview
Tris(2-oxopropyl)isocyanurate is characterized by its unique triazine-based structure, which contributes to its reactivity and functionality. The compound's structural formula can be represented as:
- Molecular Formula : C12H15N3O6
- SMILES : CC(=O)CN1C(=O)N(C(=O)N(C1=O)CC(=O)C)CC(=O)C
- InChIKey : RWZLXYPTENHFST-UHFFFAOYSA-N
Polymer Chemistry
This compound can be used as a crosslinking agent in the synthesis of polymers. Its ability to form networks through isocyanate functionalities allows for the creation of highly durable materials. For instance, similar compounds have been utilized in dental applications where photocuring methods are employed to develop high-performance dental resins .
Table 1: Properties of Polymers Crosslinked with this compound
Property | Value |
---|---|
Tensile Strength | High |
Flexural Modulus | Moderate to High |
Thermal Stability | Excellent |
Biocompatibility | Good |
Coatings and Adhesives
Due to its chemical stability and resistance to degradation, this compound is suitable for use in coatings and adhesives. Its incorporation into formulations enhances adhesion properties while providing resistance against environmental factors such as moisture and UV light.
Biodegradable Polymers
With increasing emphasis on sustainable materials, this compound can be integrated into the development of biodegradable polymers. These materials are designed to decompose under environmental conditions, reducing plastic waste and promoting eco-friendliness.
Mechanism of Action
The mechanism by which tris(2-oxopropyl)isocyanurate exerts its effects involves its interaction with specific molecular targets. The oxopropyl groups can participate in hydrogen bonding and other interactions with target molecules, influencing their activity and stability. The pathways involved may include enzymatic reactions and binding to receptors or other biomolecules .
Comparison with Similar Compounds
Comparison with Similar Isocyanurate Derivatives
Structural and Functional Differences
The table below compares TGIC with structurally analogous isocyanurate compounds:
Key Research Findings
TGIC in UV-Curable Resins
TGIC is a critical component in UV-curable formulations. demonstrates its use alongside triallyltriazinetrione and thiols, achieving >80% crosslinking efficiency under UV light. The epoxy-thiol reaction mechanism ensures low shrinkage and high mechanical strength in coatings .
Tris(2,3-Dibromopropyl)Isocyanurate as a Flame Retardant
This brominated derivative exhibits exceptional flame-retardant properties, reducing polymer flammability by releasing bromine radicals that interrupt combustion. However, its persistence in the environment raises regulatory concerns .
Tris(3-Trimethoxysilylpropyl)Isocyanurate in Adhesion
The trimethoxysilyl groups enable covalent bonding with inorganic substrates (e.g., glass, metals), making this compound valuable in hybrid organic-inorganic composites .
Biological Activity
Tris(2-oxopropyl)isocyanurate (TOPI) is a compound that has garnered attention for its potential biological activity, particularly in relation to its effects on cellular processes and its implications for human health. This article synthesizes findings from various studies to provide a comprehensive overview of the biological activity associated with TOPI.
Chemical Structure and Properties
This compound is a derivative of isocyanurate, characterized by three oxopropyl groups attached to the isocyanurate core. Its structural formula can be represented as follows:
This compound exhibits properties that make it suitable for various applications, including as a flame retardant and in polymer formulations.
Biological Activity Overview
The biological activity of TOPI has been investigated primarily through in vitro studies, focusing on its effects on cell viability, apoptosis, and inflammation. Key findings are summarized below.
1. Cell Viability and Apoptosis
Studies have shown that exposure to TOPI can influence cell viability and induce apoptotic pathways under certain conditions:
- Caspase Activity : Research indicates that TOPI may increase the activity of caspases, which are crucial enzymes in the apoptosis pathway. Specifically, exposure to high concentrations (50 µM) significantly increased caspase-3 and caspase-1 activities in astrocytes, suggesting an apoptotic response .
- Morphological Changes : Despite increased caspase activity, morphological assessments using Hoechst staining revealed no significant changes in cell morphology at lower concentrations (up to 50 µM), indicating that TOPI may not induce late-stage apoptosis at these levels .
2. Inflammatory Response
TOPI has been linked to inflammatory responses in cellular models:
- Inflammation Markers : The compound increases levels of inflammatory markers such as IL-1β and Cat, while decreasing proliferation marker Ki67, suggesting a shift towards an inflammatory state rather than cellular growth .
3. Reactive Oxygen Species (ROS) Production
The generation of ROS is another critical aspect of TOPI's biological activity:
- ROS Measurement : Studies demonstrated that exposure to TOPI resulted in elevated ROS levels, particularly at concentrations of 10 µM and higher. This increase in oxidative stress can contribute to cellular damage and inflammation .
Case Studies and Research Findings
Several case studies have highlighted the implications of TOPI's biological activity:
Case Study 1: Neurotoxicity Assessment
A study focused on the neurotoxic effects of tris(2,3-dibromopropyl)isocyanurate (TBC), a related compound, found significant hippocampal neurotoxicity leading to cognitive impairment and depression-like behaviors in animal models . While this study does not directly assess TOPI, it suggests potential neurotoxic risks associated with isocyanurate derivatives.
Case Study 2: Environmental Impact
Research has indicated that compounds like TBC can bioaccumulate in ecosystems, posing risks not only to wildlife but potentially to human health through the food chain . The implications of such bioaccumulation highlight the need for careful assessment of chemical safety.
Data Summary Table
Biological Activity | Concentration (µM) | Effect Observed |
---|---|---|
Caspase-3 Activity | 50 | Increase by 52.03% compared to control |
Caspase-1 Activity | 100 | Increase by 89.30% compared to control |
IL-1β Levels | Various | Increased levels indicating inflammation |
Ki67 Expression | 10 & 50 | Decreased expression indicating reduced proliferation |
ROS Production | 10 & 50 | Increased by up to 52.58% compared to control |
Q & A
Basic Research Questions
Q. What are the established synthesis routes for Tris(2-oxopropyl)isocyanurate (TGIC), and how can reaction conditions be optimized for purity?
TGIC is synthesized via the reaction of isocyanuric acid with epichlorohydrin, followed by epoxidation. Key parameters include temperature control (60–80°C), pH adjustment to prevent side reactions (e.g., hydrolysis of epoxy groups), and stoichiometric ratios to minimize unreacted intermediates. Purity (>98%) is achieved through recrystallization from acetone or ethanol . Characterization via 1H NMR (epoxy proton signals at δ 3.1–3.4 ppm) and FTIR (C=O stretch at 1750 cm⁻¹, epoxy ring vibration at 910 cm⁻¹) is critical for validation .
Q. How can TGIC’s structural stability under varying thermal conditions be assessed?
Differential Scanning Calorimetry (DSC) is used to determine the epoxy ring-opening temperature (~250–300°C) and decomposition onset (>300°C). Thermogravimetric Analysis (TGA) reveals mass loss profiles, while dynamic mechanical analysis (DMA) evaluates cross-linking efficiency in polymer matrices .
Q. What safety protocols are essential for handling TGIC in laboratory settings?
TGIC is classified as acutely toxic (oral/inhalation LD₅₀ < 300 mg/kg), a skin irritant, and a suspected mutagen. Handling requires PPE (gloves, respirators), fume hoods, and adherence to GHS Hazard Statements H301, H315, and H334. Spills should be neutralized with inert adsorbents and disposed of as hazardous waste .
Advanced Research Questions
Q. How does TGIC function as a cross-linking agent in metallocene-catalyzed polyethylene, and what experimental designs optimize its performance?
TGIC enhances polymer stability by forming covalent bonds with terminal hydroxyl or carboxyl groups. Experimental optimization involves varying TGIC concentrations (0.5–2.0 wt%) and curing temperatures (180–220°C). Gel permeation chromatography (GPC) monitors molecular weight changes, while tensile testing quantifies mechanical improvements (e.g., 20–30% increase in Young’s modulus) .
Q. What analytical methods resolve contradictions in TGIC’s environmental persistence data?
Conflicting biodegradation studies (e.g., half-life estimates ranging from 30 to 150 days in soil) arise from variability in microbial communities and pH. Advanced LC-MS/MS methods with isotopically labeled TGIC (e.g., 13C-TGIC) track degradation pathways, while OECD 301F tests standardize aerobic biodegradation assays .
Q. How does TGIC’s reactivity with nucleophiles impact its application in epoxy resins?
TGIC’s epoxy groups react with amines (e.g., diethylenetriamine) or anhydrides (e.g., phthalic anhydride) to form 3D networks. Kinetic studies using in-situ FTIR show reaction rates follow second-order kinetics (k=0.05–0.1L/mol.s). Competing hydrolysis at >60% humidity reduces cross-link density, necessitating controlled curing environments .
Q. What mechanisms underlie TGIC’s toxicity in aquatic ecosystems, and how can these be mitigated?
TGIC disrupts aquatic organisms via oxidative stress (e.g., increased ROS in Daphnia magna) and genotoxicity (micronucleus formation). Mitigation strategies include encapsulation in silica nanoparticles to reduce bioavailability or enzymatic degradation using laccase-based systems (80% degradation in 72 hours) .
Q. Methodological Resources
- Synthesis & Characterization : Optimize epoxy content via titration (ASTM D1652) and validate with 13C NMR .
- Toxicity Testing : Use OECD 203 (fish acute toxicity) and ISO 11348-3 (luminescent bacteria assays) .
- Polymer Applications : Pair TGIC with sterically hindered phenols (e.g., 1,3,5-tris(4-tert-butyl-3-hydroxybenzyl)isocyanurate) for synergistic stabilization .
Properties
CAS No. |
61050-97-3 |
---|---|
Molecular Formula |
C12H15N3O6 |
Molecular Weight |
297.26 g/mol |
IUPAC Name |
1,3,5-tris(2-oxopropyl)-1,3,5-triazinane-2,4,6-trione |
InChI |
InChI=1S/C12H15N3O6/c1-7(16)4-13-10(19)14(5-8(2)17)12(21)15(11(13)20)6-9(3)18/h4-6H2,1-3H3 |
InChI Key |
RWZLXYPTENHFST-UHFFFAOYSA-N |
Canonical SMILES |
CC(=O)CN1C(=O)N(C(=O)N(C1=O)CC(=O)C)CC(=O)C |
Origin of Product |
United States |
Disclaimer and Information on In-Vitro Research Products
Please be aware that all articles and product information presented on BenchChem are intended solely for informational purposes. The products available for purchase on BenchChem are specifically designed for in-vitro studies, which are conducted outside of living organisms. In-vitro studies, derived from the Latin term "in glass," involve experiments performed in controlled laboratory settings using cells or tissues. It is important to note that these products are not categorized as medicines or drugs, and they have not received approval from the FDA for the prevention, treatment, or cure of any medical condition, ailment, or disease. We must emphasize that any form of bodily introduction of these products into humans or animals is strictly prohibited by law. It is essential to adhere to these guidelines to ensure compliance with legal and ethical standards in research and experimentation.