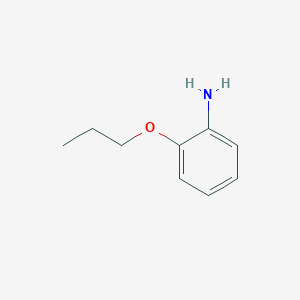
2-Propoxyaniline
Overview
Description
2-Propoxyaniline (CAS: 220594-10-5), also known as 5-nitro-2-propoxyaniline (P-4000), is a synthetic aromatic amine derivative historically recognized as one of the most potent artificial sweeteners, with a sweetness intensity approximately 4,000 times greater than sucrose . Its molecular formula is C₉H₁₃NO, and structural characterization via ¹H-NMR (DMSO-d₆) reveals peaks at δ 6.75 (d, J = 8.0 Hz), 6.62–6.67 (m), and 3.87 (t, J = 6.6 Hz), confirming the propoxy group and aromatic amine functionalities . Despite its high sweetness potency, this compound was banned due to unresolved toxicity concerns, though its exact mechanism of action on taste receptors (T1R2/T1R3 heterodimer) remains understudied .
Preparation Methods
Alkylation of 2-Aminophenol with Propyl Halides
Base-Mediated Propoxylation
The direct alkylation of 2-aminophenol using propyl bromide in the presence of sodium hydride (NaH) is a widely adopted method. This reaction proceeds via nucleophilic substitution, where the phenolic oxygen is deprotonated to form an alkoxide, which subsequently displaces the halide.
Procedure :
- Reactants : 2-Aminophenol (1.0 equiv), propyl bromide (1.2 equiv), NaH (1.5 equiv).
- Solvent : Anhydrous acetonitrile.
- Conditions : Room temperature, 24 hours under inert atmosphere.
- Yield : 70–80% after purification by column chromatography.
Mechanistic Insights :
The reaction’s efficiency hinges on the base’s ability to deprotonate the hydroxyl group, facilitating SN2 displacement. Steric hindrance from the ortho-amino group slightly reduces reactivity compared to para-substituted analogs.
Industrial Adaptation :
Continuous flow reactors enhance safety and scalability, achieving 85% yield at 50°C with reduced reaction time (4 hours).
Ullmann-Type Coupling for Direct Synthesis
Copper-Catalyzed Arylation
Ullmann coupling between 2-bromophenol and propylamine offers a one-step route to 2-propoxy aniline, leveraging copper catalysts to form the C–O bond.
Procedure :
- Catalyst : CuI (10 mol%), 1,10-phenanthroline (20 mol%).
- Base : Cs₂CO₃ (2.0 equiv).
- Solvent : Dimethylformamide (DMF).
- Conditions : 110°C, 12 hours.
- Yield : 65–70% after distillation.
Optimization Data :
Parameter | Optimal Value | Yield Impact |
---|---|---|
Catalyst Loading | 10 mol% CuI | Max yield |
Temperature | 110°C | <5% drop at 100°C |
Solvent Polarity | High (DMF) | 20% drop in toluene |
Limitations :
Over-reduction of the aryl bromide and homocoupling byproducts necessitate careful stoichiometric control.
Reductive Amination of Nitro Precursors
Hydrogenation of 2-Nitro-4-propoxyphenol
This two-step method involves propoxylation followed by nitro group reduction.
Step 1: Nitrophenol Alkylation
- Reactants : 4-Amino-3-nitrophenol, 1-bromopropane, LiOH·H₂O.
- Solvent : Ethanol.
- Conditions : 70°C, 12 hours.
- Yield : 80% after recrystallization.
Step 2: Catalytic Hydrogenation
- Catalyst : Pd/C (5 wt%).
- Conditions : H₂ (1 atm), 25°C, 6 hours.
- Yield : 95% conversion to 2-propoxy aniline.
Comparative Efficiency :
Method | Steps | Total Yield | Cost (Relative) |
---|---|---|---|
Direct Alkylation | 1 | 70% | $ |
Reductive Amination | 2 | 76% | $$ |
Nucleophilic Substitution of Aniline Derivatives
Propylation of Chloroaniline
Reacting 2-chloroaniline with sodium propoxide under high-temperature conditions achieves propoxy substitution.
Procedure :
- Reactants : 2-Chloroaniline (1.0 equiv), NaOPr (1.5 equiv).
- Solvent : Diglyme.
- Conditions : 150°C, 8 hours.
- Yield : 60% with 90% purity.
Challenges :
Competing hydrolysis of sodium propoxide and elimination side reactions necessitate anhydrous conditions.
Industrial-Scale Production and Optimization
Continuous Flow Synthesis
Adoption of microreactor technology improves heat transfer and mixing:
- Residence Time : 30 minutes.
- Throughput : 1.2 kg/hr.
- Purity : >99% by HPLC.
Chemical Reactions Analysis
Types of Reactions
2-Propoxyaniline undergoes various chemical reactions, including:
Oxidation: It can be oxidized to form corresponding quinone derivatives.
Reduction: The compound can be further reduced to form more saturated amine derivatives.
Substitution: Electrophilic substitution reactions can occur on the benzene ring, such as nitration, sulfonation, and halogenation.
Common Reagents and Conditions
Oxidation: Common oxidizing agents include potassium permanganate (KMnO4) and chromium trioxide (CrO3).
Reduction: Hydrogen gas (H2) in the presence of palladium on carbon (Pd/C) is typically used.
Major Products Formed
Oxidation: Quinone derivatives.
Reduction: Saturated amine derivatives.
Substitution: Nitro, sulfonyl, and halogenated derivatives.
Scientific Research Applications
Investigations into Biological Interactions
Research has explored the interactions of 2-propoxyaniline with biological molecules. Studies indicate that it can act as a model compound for examining how small organic molecules interact with biomolecules, particularly in the context of sweet taste perception. Its derivatives are being studied for their potential therapeutic applications due to their biological activity.
Case Study: Sweetness Perception
A significant focus has been on the compound's interaction with sweet taste receptors. For instance, derivatives of this compound have been synthesized to investigate their binding affinity to these receptors, which is crucial for understanding sweetness mechanisms.
- Research Findings : The structural characteristics of this compound derivatives influence their sweetness intensity and receptor binding properties. This relationship is vital for developing new artificial sweeteners with improved safety profiles.
Industrial Applications
Historical Use as an Artificial Sweetener
Historically, this compound was explored as an artificial sweetener due to its high sweetness intensity compared to sucrose. However, concerns regarding its toxicity led to regulatory restrictions on its use in food products.
Application | Status |
---|---|
Artificial Sweetener | Banned due to toxicity |
Current Research Focus | Safe alternatives |
Toxicological Studies
Safety Assessments
Toxicological assessments have been conducted to evaluate the safety profile of this compound and its derivatives. Studies have shown that while some compounds exhibit significant biological activity, they may also pose health risks if consumed in large quantities.
- Key Findings : The compound has been associated with mutagenicity and potential carcinogenicity, highlighting the need for careful evaluation in any future applications.
Mechanism of Action
The mechanism of action of 2-Propoxyaniline involves its interaction with specific molecular targets. For instance, in biological systems, it may interact with enzymes or receptors, leading to modulation of biochemical pathways. The exact molecular targets and pathways can vary depending on the specific application and context .
Comparison with Similar Compounds
Comparison with Structurally Similar Compounds
Saccharin (CAS: 81-07-2)
- Structural Features : Saccharin contains a sulfonamide group instead of the propoxy-aniline backbone.
- Sweetness and Bioactivity : Saccharin’s sweetness is ~300× sucrose, significantly lower than 2-propoxyaniline. Substitutions at the 5- and 6-positions of its benzene ring minimally affect activity, unlike the critical nitro group at the 5-position in this compound .
- Toxicity: Saccharin’s carcinogenic risk in rodents is well-documented but considered negligible in humans, whereas this compound’s toxicity mechanisms (e.g., metabolic byproducts) remain uncharacterized .
5-Azido-2-propoxyaniline (Compound 6)
- Structural Modification : The nitro group in this compound is replaced with an azide group at the 5-position.
- Bioactivity : Retains sweetness comparable to sucrose in cell-based assays (hT1R2-hT1R3 HEK293T cells), confirming the 5-position’s tolerance for substitutions without activity loss .
- Utility : Used as a photoaffinity probe to map ligand-receptor interactions, unlike the parent compound .
5-(Trifluoromethyldiazirinyl)-2-propoxyaniline (Compound 22)
- Structural Features : Incorporates a trifluoromethyldiazirine photophore at the 5-position.
- Bioactivity : Exhibits sweetness equivalent to sucrose, demonstrating that bulky substituents at the 5-position are compatible with receptor binding .
- Synthesis: Achieved via MnO₂ oxidation of diaziridine intermediates, yielding 94% purity .
5-Benzoyl-2-propoxyaniline (Compound 10)
- Structural Features: A benzophenone group replaces the nitro moiety.
5-Methyl-2-propoxyaniline
- Structural Features : A methyl group substitutes the nitro group.
- Properties: Retains solubility in organic solvents (e.g., ethanol) but lacks sweetness data, suggesting reduced receptor affinity compared to this compound .
Functional Comparison with Other Sweeteners
Compound | Sweetness (vs. Sucrose) | Key Functional Group | Toxicity Status | Application |
---|---|---|---|---|
This compound | 4,000× | Nitro-aniline | Banned | Historic sweetener |
Saccharin | 300× | Sulfonamide | Approved (with limits) | Food additive |
5-Azido-2-propoxyaniline | 1× (sucrose-equivalent) | Azide | Research-only | Photoaffinity labeling |
Aspartame | 200× | Dipeptide methyl ester | Approved | Diet products |
Mechanistic Insights
- Receptor Binding : The 5-position in this compound is critical for hydrogen bonding with T1R3’s Venus flytrap domain. Derivatives with photophores (e.g., azide, diazirine) retain binding affinity, enabling receptor mapping .
- Toxicity: Proposed mechanisms include nitro group reduction to carcinogenic aryl amines or interference with metabolic pathways, though conclusive data are lacking .
Biological Activity
2-Propoxyaniline, a substituted aniline compound, has garnered attention in various fields of biological research due to its potential antimicrobial and cytotoxic properties. This article reviews the biological activity of this compound, focusing on its synthesis, antimicrobial efficacy, mechanism of action, and cytotoxicity, supported by recent studies and case analyses.
Chemical Structure and Synthesis
This compound is characterized by the presence of a propoxy group attached to the aniline ring. Its synthesis typically involves the alkylation of aniline with propyl alcohol under acidic conditions. The resulting compound serves as a precursor for various derivatives that enhance biological activity.
Antimicrobial Properties
Recent studies have highlighted the antimicrobial efficacy of this compound and its derivatives against various pathogens. Notably, compounds derived from this compound have shown significant activity against methicillin-resistant Staphylococcus aureus (MRSA) and Mycobacterium tuberculosis.
Table 1: Antimicrobial Activity of this compound Derivatives
Compound | MIC (µM) | Target Pathogen |
---|---|---|
3-Hydroxy-N-(2-propoxyphenyl)naphthalene-2-carboxamide | 12 | MRSA |
N-[2-(but-2-yloxy)-phenyl]-3-hydroxynaphthalene-2-carboxamide | 23 | M. tuberculosis |
N-[2-(but-2-yloxy)-phenyl]-3-hydroxynaphthalene-2-carboxamide | <12 | M. avium subsp. paratuberculosis |
The minimum inhibitory concentration (MIC) values indicate that certain derivatives of this compound exhibit enhanced potency compared to standard antibiotics such as ampicillin and rifampicin .
The mechanism by which this compound exerts its antimicrobial effects is believed to involve interference with bacterial cell metabolism and energy production. It may inhibit key enzymes involved in cell wall synthesis or disrupt electron transport chains within bacterial cells, leading to cell death. For instance, studies suggest that these compounds may act on mycobacterial proton pumps or inhibit amino acid biosynthesis .
Cytotoxicity Studies
Cytotoxicity assessments using human cell lines have shown that while some derivatives possess strong antibacterial properties, they exhibit low cytotoxicity towards normal human dermal fibroblasts (NHDF). This is crucial for therapeutic applications as it indicates a favorable safety profile.
Table 2: Cytotoxicity of Selected Compounds
Compound | IC50 (µM) | Cell Line |
---|---|---|
3-Hydroxy-N-(2-propoxyphenyl)naphthalene-2-carboxamide | >100 | NHDF |
N-[2-(but-2-yloxy)-phenyl]-3-hydroxynaphthalene-2-carboxamide | >100 | NHDF |
The high IC50 values suggest that these compounds can be effective against pathogens without significantly harming human cells .
Case Studies
In one notable study, researchers synthesized various alkoxy derivatives of this compound and evaluated their antibacterial activities against clinical isolates of MRSA. The findings revealed that certain derivatives exhibited up to four times greater efficacy than conventional antibiotics, highlighting their potential as alternative therapeutic agents .
Another investigation focused on the impact of these compounds on photosynthetic electron transport in chloroplasts. Results indicated substantial inhibition of electron transport, suggesting possible applications in herbicide development or further exploration in plant biology .
Q & A
Basic Research Questions
Q. What are the optimal synthetic pathways for 2-Propoxyaniline derivatives, and how do reaction conditions influence yield and purity?
- Methodological Answer : The synthesis of this compound derivatives often involves nucleophilic substitution or esterification reactions. Key variables include temperature (e.g., 60–80°C for amide bond formation), solvent polarity (e.g., dimethylformamide vs. ethanol), and catalyst selection (e.g., triethylamine for deprotonation). Yield optimization requires monitoring reaction kinetics via HPLC or GC-MS, while purity is assessed using NMR and elemental analysis . For photoreactive analogs, UV irradiation protocols must balance activation efficiency with compound stability .
Q. Which analytical techniques are most reliable for characterizing this compound’s structural and functional properties?
- Methodological Answer : Combine spectroscopic methods:
- FT-IR : Confirm functional groups (e.g., -NH₂ at ~3400 cm⁻¹, ether C-O-C at ~1250 cm⁻¹).
- NMR : Assign proton environments (e.g., aromatic protons at δ 6.5–7.5 ppm, propoxy methyl groups at δ 1.0–1.5 ppm).
- Mass Spectrometry : Validate molecular weight and fragmentation patterns.
Cross-validate results with computational simulations (e.g., Gaussian for vibrational frequencies) to address discrepancies .
Q. How can researchers ensure reproducibility in this compound-based assays, particularly in sweet taste receptor studies?
- Methodological Answer : Standardize cell-based assays using HEK293-T1R2/T1R3 transfected cells. Control variables:
- Ligand concentration (e.g., 0.1–10 μM ranges).
- Buffer composition (pH 7.4 to mimic physiological conditions).
- Blind protocols to minimize observer bias.
Replicate experiments across independent labs and share raw data via repositories like Zenodo to enhance transparency .
Advanced Research Questions
Q. What molecular docking strategies elucidate this compound’s binding affinity to sweet taste receptors, and how do steric effects alter activity?
- Methodological Answer : Use AutoDock Vina or Schrödinger Suite for docking simulations. Key steps:
- Receptor Preparation : Homology model T1R2/T1R3 subunits based on class C GPCR templates.
- Ligand Flexibility : Account for propoxy chain conformers via Monte Carlo sampling.
- Validation : Compare docking scores (e.g., ΔG values) with experimental EC₅₀ data. Bulky substituents (e.g., benzoyl groups) reduce activity by ~90%, highlighting steric clashes in the receptor’s Venus flytrap domain .
Q. How can conflicting data on this compound’s metabolic stability be resolved across in vitro and in vivo models?
- Methodological Answer : Apply tiered testing:
- In Vitro : Liver microsomal assays (human vs. rodent) to assess CYP450-mediated degradation.
- In Silico : Predict metabolites using software like Meteor (Lhasa Limited).
- In Vivo : Pharmacokinetic studies in Sprague-Dawley rats with LC-MS/MS plasma analysis. Discrepancies often arise from interspecies metabolic differences or sampling timepoints; use mixed-effects modeling to adjust for variability .
Q. What strategies mitigate batch-to-batch variability in this compound synthesis for longitudinal studies?
- Methodological Answer : Implement quality-by-design (QbD) principles:
- Critical Quality Attributes (CQAs) : Define purity (>98%), residual solvent levels (<0.1%).
- Process Parameters : Optimize stirring rate (e.g., 500 rpm for homogeneity) and cooling gradients.
- Statistical Control : Use multivariate analysis (e.g., PCA) to identify outlier batches and refine SOPs .
Q. Methodological & Ethical Considerations
Q. How should researchers design studies to address potential neurotoxic effects of this compound derivatives?
- Methodological Answer : Follow OECD guidelines for neurotoxicity screening:
- In Vitro : SH-SY5Y cell viability assays with ROS measurement.
- In Vivo : Open-field and Morris water maze tests in rodents.
- Ethical Compliance : Obtain IACUC approval, minimize animal cohorts via power analysis, and adhere to ARRIVE reporting standards .
Q. What frameworks (e.g., FINER, PICO) are suitable for formulating hypotheses about this compound’s structure-activity relationships?
- Methodological Answer : Apply the FINER criteria :
- Feasible : Ensure access to synthetic intermediates and analytical facilities.
- Novel : Explore understudied substituents (e.g., fluorinated propoxy chains).
- Ethical : Disclose conflicts of interest if collaborating with flavorant manufacturers.
Use PICO for clinical relevance: Population (human taste perception), Intervention (this compound dosage), Comparison (sucrose), Outcome (sweetness intensity) .
Q. Data Integrity & Reporting
Q. How can researchers validate unexpected results, such as anomalous sweetness potency in this compound analogs?
- Methodological Answer : Conduct triplicate assays with blinded positive/negative controls. Employ orthogonal methods:
- Calorimetry : Measure ligand-receptor binding enthalpy.
- Patch-Clamp Electrophysiology : Confirm ion channel activation in taste cells.
Report negative results transparently to avoid publication bias .
Q. What are the best practices for archiving and sharing this compound research data to meet NIH guidelines?
- Methodological Answer :
- Raw Data : Deposit spectra, chromatograms, and assay readouts in Figshare or ICPSR.
- Metadata : Annotate synthetic procedures using ISA-TAB format.
- Retention : Maintain lab notebooks and electronic records for 10+ years.
Include a Data Availability Statement in manuscripts, specifying embargo periods if applicable .
Properties
IUPAC Name |
2-propoxyaniline | |
---|---|---|
Source | PubChem | |
URL | https://pubchem.ncbi.nlm.nih.gov | |
Description | Data deposited in or computed by PubChem | |
InChI |
InChI=1S/C9H13NO/c1-2-7-11-9-6-4-3-5-8(9)10/h3-6H,2,7,10H2,1H3 | |
Source | PubChem | |
URL | https://pubchem.ncbi.nlm.nih.gov | |
Description | Data deposited in or computed by PubChem | |
InChI Key |
AXXYMKMFXNCXGM-UHFFFAOYSA-N | |
Source | PubChem | |
URL | https://pubchem.ncbi.nlm.nih.gov | |
Description | Data deposited in or computed by PubChem | |
Canonical SMILES |
CCCOC1=CC=CC=C1N | |
Source | PubChem | |
URL | https://pubchem.ncbi.nlm.nih.gov | |
Description | Data deposited in or computed by PubChem | |
Molecular Formula |
C9H13NO | |
Source | PubChem | |
URL | https://pubchem.ncbi.nlm.nih.gov | |
Description | Data deposited in or computed by PubChem | |
DSSTOX Substance ID |
DTXSID70366584 | |
Record name | 2-propoxyaniline | |
Source | EPA DSSTox | |
URL | https://comptox.epa.gov/dashboard/DTXSID70366584 | |
Description | DSSTox provides a high quality public chemistry resource for supporting improved predictive toxicology. | |
Molecular Weight |
151.21 g/mol | |
Source | PubChem | |
URL | https://pubchem.ncbi.nlm.nih.gov | |
Description | Data deposited in or computed by PubChem | |
CAS No. |
4469-78-7 | |
Record name | 2-propoxyaniline | |
Source | EPA DSSTox | |
URL | https://comptox.epa.gov/dashboard/DTXSID70366584 | |
Description | DSSTox provides a high quality public chemistry resource for supporting improved predictive toxicology. | |
Record name | 4469-78-7 | |
Source | European Chemicals Agency (ECHA) | |
URL | https://echa.europa.eu/information-on-chemicals | |
Description | The European Chemicals Agency (ECHA) is an agency of the European Union which is the driving force among regulatory authorities in implementing the EU's groundbreaking chemicals legislation for the benefit of human health and the environment as well as for innovation and competitiveness. | |
Explanation | Use of the information, documents and data from the ECHA website is subject to the terms and conditions of this Legal Notice, and subject to other binding limitations provided for under applicable law, the information, documents and data made available on the ECHA website may be reproduced, distributed and/or used, totally or in part, for non-commercial purposes provided that ECHA is acknowledged as the source: "Source: European Chemicals Agency, http://echa.europa.eu/". Such acknowledgement must be included in each copy of the material. ECHA permits and encourages organisations and individuals to create links to the ECHA website under the following cumulative conditions: Links can only be made to webpages that provide a link to the Legal Notice page. | |
Synthesis routes and methods I
Procedure details
Synthesis routes and methods II
Procedure details
Retrosynthesis Analysis
AI-Powered Synthesis Planning: Our tool employs the Template_relevance Pistachio, Template_relevance Bkms_metabolic, Template_relevance Pistachio_ringbreaker, Template_relevance Reaxys, Template_relevance Reaxys_biocatalysis model, leveraging a vast database of chemical reactions to predict feasible synthetic routes.
One-Step Synthesis Focus: Specifically designed for one-step synthesis, it provides concise and direct routes for your target compounds, streamlining the synthesis process.
Accurate Predictions: Utilizing the extensive PISTACHIO, BKMS_METABOLIC, PISTACHIO_RINGBREAKER, REAXYS, REAXYS_BIOCATALYSIS database, our tool offers high-accuracy predictions, reflecting the latest in chemical research and data.
Strategy Settings
Precursor scoring | Relevance Heuristic |
---|---|
Min. plausibility | 0.01 |
Model | Template_relevance |
Template Set | Pistachio/Bkms_metabolic/Pistachio_ringbreaker/Reaxys/Reaxys_biocatalysis |
Top-N result to add to graph | 6 |
Feasible Synthetic Routes
Disclaimer and Information on In-Vitro Research Products
Please be aware that all articles and product information presented on BenchChem are intended solely for informational purposes. The products available for purchase on BenchChem are specifically designed for in-vitro studies, which are conducted outside of living organisms. In-vitro studies, derived from the Latin term "in glass," involve experiments performed in controlled laboratory settings using cells or tissues. It is important to note that these products are not categorized as medicines or drugs, and they have not received approval from the FDA for the prevention, treatment, or cure of any medical condition, ailment, or disease. We must emphasize that any form of bodily introduction of these products into humans or animals is strictly prohibited by law. It is essential to adhere to these guidelines to ensure compliance with legal and ethical standards in research and experimentation.