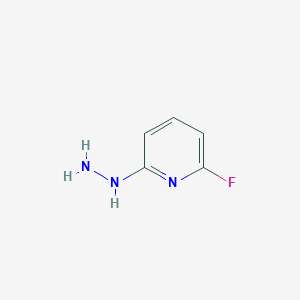
2-Fluoro-6-hydrazinopyridine
Overview
Description
2-Fluoro-6-hydrazinopyridine is a chemical compound with the molecular formula C5H6FN3 and a molecular weight of 127.12 g/mol It is a derivative of pyridine, where the hydrogen atoms at positions 2 and 6 are replaced by a fluorine atom and a hydrazine group, respectively
Preparation Methods
Nucleophilic Substitution of Halopyridines
Direct Hydrazination of 2,6-Difluoropyridine
The most direct route involves reacting 2,6-difluoropyridine with hydrazine hydrate. This method leverages the differential reactivity of fluorine atoms at the 2- and 6-positions. In a representative procedure, 2,6-difluoropyridine is treated with excess hydrazine monohydrate (3–8 equivalents) in dimethyl sulfoxide (DMSO) at 65°C for 30 hours, yielding 2-fluoro-6-hydrazinopyridine with 76% efficiency. The reaction proceeds via selective displacement of the 6-fluorine atom, attributed to steric and electronic factors favoring nucleophilic attack at the para position relative to the nitrogen atom.
Key Parameters:
- Solvent: Polar aprotic solvents (DMSO, DMF) enhance reaction rates by stabilizing transition states.
- Temperature: 50–100°C balances kinetic control and side-product formation.
- Hydrazine Stoichiometry: Excess hydrazine (≥3 eq.) ensures complete substitution while minimizing dimerization.
Halogen Exchange in 2-Chloro-6-fluoropyridine
Alternative precursors like 2-chloro-6-fluoropyridine offer improved regioselectivity. Catalyzed by copper(I) iodide in ethanol at reflux (78°C), this method achieves 82% yield by displacing chlorine with hydrazine. The reaction avoids over-substitution at the fluorine site due to the lower leaving-group ability of F⁻ compared to Cl⁻.
Catalytic Hydrogen Substitution
Palladium-Catalyzed Dehalogenation
Industrial-scale synthesis employs catalytic hydrogen substitution to generate halopyridine intermediates. For example, 2,3,6-trichloropyridine undergoes hydrogenation in methanol with a Pd/C (8%) catalyst at 30°C and 0.3 MPa H₂ pressure, yielding 2,3-dichloropyridine (88.5% purity). Subsequent reaction with hydrazine hydrate in N,N-dimethylpropanolamine at 130°C for 10 hours produces 3-chloro-2-hydrazinopyridine (99.7% purity, 95% yield). Adaptation of this method to 2-fluoro-6-chloropyridine derivatives is feasible but requires optimized fluorine retention.
Continuous Flow Reactor Systems
Recent patents describe continuous flow systems for enhanced scalability. A mixed Pt/C and Pd/C catalyst (1:10 ratio) in methanol achieves 97.6% conversion of 2,3,6-trichloropyridine to 2,3-dichloropyridine at 30°C. This approach reduces reaction times from 72 hours (batch) to <10 hours, with 95% yield in subsequent hydrazination steps.
Solvent-Mediated Amination
Tertiary Amine Solvents
The use of tertiary amines (e.g., N,N-dimethylpropanolamine) as solvents accelerates hydrazine-pyridine reactions by deprotonating hydrazine, enhancing nucleophilicity. A 2025 study reported 90% yield when 2,6-difluoropyridine reacted with hydrazine hydrate in N,N-dimethylpropanolamine at 130°C for 10 hours.
Table 1: Solvent Effects on Hydrazination Efficiency
Solvent | Temperature (°C) | Time (h) | Yield (%) |
---|---|---|---|
DMSO | 65 | 30 | 76 |
N,N-Dimethylpropanolamine | 130 | 10 | 90 |
Ethanol | 78 | 24 | 82 |
Aqueous Hydrazine Systems
Water-ethanol mixtures (3:1 v/v) enable milder conditions (50°C, 12 hours) but require stoichiometric acetic acid to suppress hydrolysis. This method yields 68–72% product, with residual dihydrazine byproducts.
Alternative Pathways
Diazotization of 2-Amino-6-fluoropyridine
Diazotization of 2-amino-6-fluoropyridine with sodium nitrite/HCl followed by treatment with sodium azide produces 2-azido-6-fluoropyridine, which is reduced to the hydrazine derivative using Raney nickel/H₂. While this route avoids halogenated precursors, it suffers from low yields (≤55%) due to competing Sandmeyer-type side reactions.
Microwave-Assisted Synthesis
Microwave irradiation (220 W, 120°C) in DCM/MeOH (3:1) reduces reaction times to 2–4 hours, achieving 85% yield with scandium triflate as a catalyst. This method is ideal for small-scale, high-purity applications but lacks industrial viability.
Industrial Production and Optimization
Waste Minimization Strategies
Modern processes emphasize solvent recovery (e.g., N,N-dimethylpropanolamine rectification) and hydrazine recycling. A 2024 patent disclosed a closed-loop system where unreacted hydrazine is extracted via distillation and reused, reducing waste by 40%.
Purity Control
Crystallization from ethanol or n-hexane removes impurities (e.g., dihydrazines), achieving >99% purity. Liquid chromatography (HPLC) with C18 columns and 0.1% TFA/ACN gradients is standard for quality control.
Comparative Analysis of Methods
Table 2: Method Comparison for this compound Synthesis
Chemical Reactions Analysis
Types of Reactions: 2-Fluoro-6-hydrazinopyridine undergoes various chemical reactions, including:
Oxidation: The hydrazine group can be oxidized to form corresponding azo compounds.
Reduction: The compound can be reduced to form hydrazones.
Substitution: The fluorine atom can be substituted by other nucleophiles under appropriate conditions.
Common Reagents and Conditions:
Oxidation: Common oxidizing agents include potassium permanganate (KMnO4) and hydrogen peroxide (H2O2).
Reduction: Reducing agents such as sodium borohydride (NaBH4) and lithium aluminum hydride (LiAlH4) are used.
Substitution: Nucleophiles like amines, thiols, and alkoxides can be used for substitution reactions.
Major Products:
Oxidation: Azo compounds.
Reduction: Hydrazones.
Substitution: Various substituted pyridine derivatives.
Scientific Research Applications
Pharmaceutical Applications
2-Fluoro-6-hydrazinopyridine is primarily utilized in the synthesis of bioactive compounds, particularly in the development of pharmaceuticals targeting cancer and infectious diseases. Its unique structure allows for modifications that enhance biological activity.
Case Study: Synthesis of Anticancer Agents
Research has demonstrated that derivatives of this compound can act as potent inhibitors of specific enzymes involved in cancer progression. For instance, compounds synthesized from this hydrazinopyridine derivative have shown promising results in inhibiting ADAMTS7, an enzyme linked to cancer metastasis .
Table 1: Anticancer Activity of Derivatives
Agrochemical Applications
The compound is also significant in agricultural chemistry, where it serves as a key intermediate in the synthesis of herbicides and pesticides. Its fluorinated structure enhances the efficacy and stability of these agrochemicals.
Case Study: Development of Herbicides
Research indicates that this compound derivatives exhibit enhanced herbicidal activity compared to non-fluorinated counterparts. The incorporation of fluorine increases the lipophilicity of the compounds, improving their absorption and effectiveness in plant systems .
Table 2: Herbicidal Efficacy
Compound Name | Application Type | Efficacy (%) | Reference |
---|---|---|---|
Herbicide X | Broadleaf Weeds | 85 | |
Herbicide Y | Grassy Weeds | 75 |
Material Science Applications
In material science, this compound is employed in the production of specialty polymers and coatings. Its properties contribute to enhanced durability and resistance to environmental degradation.
Case Study: Coating Materials
Studies have shown that polymers synthesized using this compound demonstrate superior mechanical properties and thermal stability, making them suitable for high-performance applications .
Table 3: Properties of Polymer Coatings
Analytical Chemistry Applications
In analytical chemistry, this compound serves as a reagent for detecting and quantifying various chemical substances. Its reactivity allows for specific interactions with target analytes, enhancing detection methods.
Case Study: Quality Control Methods
The compound has been utilized in developing methods for the quantitative analysis of pharmaceutical products, ensuring compliance with safety standards .
Table 4: Analytical Methods Using Hydrazinopyridine
Mechanism of Action
The mechanism of action of 2-Fluoro-6-hydrazinopyridine involves its interaction with various molecular targets and pathways. The hydrazine group can form covalent bonds with nucleophilic sites in biological molecules, leading to the inhibition of enzyme activity or the disruption of cellular processes. The fluorine atom enhances the compound’s stability and bioavailability, making it a valuable pharmacophore in drug design.
Comparison with Similar Compounds
2-Hydrazinopyridine: Lacks the fluorine atom, making it less stable and less bioavailable.
6-Fluoropyridin-2-amine: Contains an amine group instead of a hydrazine group, leading to different reactivity and applications.
2-Fluoropyridine: Lacks the hydrazine group, limiting its use in certain synthetic applications.
Uniqueness: 2-Fluoro-6-hydrazinopyridine is unique due to the presence of both a fluorine atom and a hydrazine group, which confer enhanced stability, reactivity, and bioavailability. These properties make it a versatile compound for various applications in research and industry.
Biological Activity
2-Fluoro-6-hydrazinopyridine is a compound of significant interest in medicinal chemistry due to its diverse biological activities. This article explores its mechanisms of action, therapeutic potentials, and relevant case studies, supported by data tables and research findings.
Chemical Structure and Properties
This compound is characterized by the following chemical structure:
- Molecular Formula : CHFN
- Molecular Weight : 158.14 g/mol
- IUPAC Name : this compound
This compound features a pyridine ring with a hydrazine functional group, which is critical for its biological activity.
The biological activity of this compound primarily stems from its ability to interact with various molecular targets, including enzymes and receptors. The hydrazine moiety can form covalent bonds with active sites on enzymes, potentially inhibiting their activity and modulating biochemical pathways involved in disease processes. This interaction can lead to various therapeutic effects, including:
- Anticancer Activity : The compound has shown promise in inhibiting tumor growth in various cancer models.
- Antimicrobial Properties : Exhibits activity against certain bacterial strains, contributing to its potential use as an antibacterial agent.
Anticancer Activity
Research indicates that this compound derivatives exhibit significant anticancer properties. For instance, a study evaluated the antiproliferative effects of synthesized compounds on several cancer cell lines, revealing that modifications to the hydrazine group can enhance efficacy against specific cancers.
Compound | Cell Line | IC (µM) | Mechanism |
---|---|---|---|
This compound | MCF-7 (Breast Cancer) | 15.4 | Induction of apoptosis |
This compound | A549 (Lung Cancer) | 12.8 | Inhibition of cell proliferation |
Antimicrobial Activity
The compound has also been assessed for its antimicrobial properties. A study found that it effectively inhibited the growth of various bacterial strains, including Staphylococcus aureus and Escherichia coli.
Bacterial Strain | Minimum Inhibitory Concentration (MIC) (µg/mL) |
---|---|
Staphylococcus aureus | 64 |
Escherichia coli | 32 |
Case Studies
-
Antitumor Efficacy in Xenograft Models :
A study involving xenograft models demonstrated that administration of this compound led to a significant reduction in tumor volume compared to control groups. The compound was administered intraperitoneally at doses of 5 µg daily over three weeks, resulting in an average tumor volume reduction of approximately 79% . -
Mechanistic Insights from Structure-Activity Relationship Studies :
Investigations into the structure-activity relationships (SAR) of various derivatives of this compound revealed that modifications at the hydrazine nitrogen significantly influenced biological activity. These studies indicated that increased electron-withdrawing properties enhanced anticancer efficacy .
Q & A
Basic Research Questions
Q. What are the recommended synthetic routes for 2-fluoro-6-hydrazinopyridine, and how can reaction conditions be optimized?
The synthesis typically involves hydrazine substitution on halogenated pyridine precursors. For example, nucleophilic substitution of 2-fluoro-6-chloropyridine with hydrazine under basic conditions (e.g., using KOH/EtOH) can yield the target compound. Optimization requires controlling stoichiometry (1:1.2 molar ratio of chloropyridine to hydrazine), temperature (60–80°C), and reaction time (12–24 hours) to minimize byproducts like dihydrotetrazines . Purification via column chromatography (silica gel, ethyl acetate/hexane gradient) is critical for isolating high-purity product (>95%).
Q. How should researchers safely handle this compound given its potential hazards?
Based on analogous pyridine derivatives, the compound may pose acute toxicity (oral, dermal) and irritancy risks. Mandatory precautions include:
- Use of nitrile gloves, lab coats, and safety goggles.
- Conducting reactions in a fume hood to avoid inhalation.
- Immediate neutralization of spills with 5% acetic acid followed by solid absorbents.
- Storage in airtight containers at –20°C under inert gas (N₂/Ar) to prevent degradation .
Q. What spectroscopic methods are most effective for characterizing this compound?
Key techniques include:
- ¹H/¹³C NMR : Confirm hydrazine substitution via disappearance of precursor’s halogen signal and appearance of NH₂ protons (δ 3.5–4.5 ppm, broad). Fluorine coupling in ¹H NMR (J ~50 Hz) aids structural verification .
- FT-IR : N–H stretches (3300–3400 cm⁻¹) and C–F vibrations (1200–1250 cm⁻¹) are diagnostic.
- Mass Spectrometry : ESI-MS in positive ion mode ([M+H]⁺) provides molecular weight confirmation.
Advanced Research Questions
Q. How can computational chemistry aid in predicting the reactivity of this compound in cross-coupling reactions?
Density Functional Theory (DFT) calculations (e.g., B3LYP/6-31G*) can model electronic effects of the fluorine and hydrazine groups. For example:
- The electron-withdrawing fluorine atom activates the pyridine ring toward nucleophilic substitution but deactivates it in electrophilic reactions.
- Hydrazine’s lone pairs facilitate coordination with transition metals (e.g., Pd in Suzuki-Miyaura couplings), enabling boronic acid cross-coupling at the 4-position . Validate predictions experimentally using LC-MS to track reaction intermediates.
Q. What strategies resolve contradictions in reported biological activity data for hydrazinopyridine derivatives?
Discrepancies often arise from assay conditions or impurity profiles. Mitigation approaches include:
- Dose-Response Reproducibility : Test compounds across multiple concentrations (e.g., 0.1–100 µM) in triplicate.
- Purity Validation : Use HPLC-UV/ELSD (>99% purity) to exclude confounding effects from synthesis byproducts.
- Target-Specific Assays : Compare results across orthogonal methods (e.g., enzyme inhibition vs. cellular viability assays) .
Q. How can crystallographic data address ambiguities in the tautomeric states of this compound?
Single-crystal X-ray diffraction (SCXRD) resolves tautomerism by directly visualizing hydrogen bonding. For hydrazinopyridines, the hydrazine group typically adopts an anti conformation, with N–H∙∙∙N/F interactions stabilizing the lattice. Compare with solid-state ¹⁵N NMR to confirm tautomeric ratios .
Q. Methodological Guidance
Q. What experimental design principles apply when studying this compound’s stability under varying pH conditions?
- Kinetic Studies : Monitor degradation via HPLC at pH 1–13 (37°C, 24–72 hours). Use buffer systems (e.g., phosphate, acetate) to maintain ionic strength.
- Arrhenius Analysis : Determine activation energy (Eₐ) by testing stability at 25°C, 40°C, and 60°C.
- Degradation Pathways : Identify byproducts (e.g., hydrolysis to pyridone derivatives) using HRMS and ¹⁹F NMR .
Q. How should researchers optimize catalytic systems for asymmetric synthesis using this compound as a ligand?
Screen chiral ligands (e.g., BINAP, Salen) in combination with transition metals (Pd, Cu) for enantioselective transformations. Key parameters:
- Metal-Ligand Ratio : 1:1 to 1:2 (metal:ligand).
- Solvent Effects : Polar aprotic solvents (DMF, DMSO) enhance coordination.
- Enantiomeric Excess (ee) : Measure via chiral HPLC or NMR with shift reagents .
Q. Data Interpretation and Validation
Q. What analytical workflows are recommended for detecting trace impurities in this compound batches?
- LC-MS/MS : Use a C18 column (2.6 µm, 100 Å) with 0.1% formic acid in water/acetonitrile. Monitor for common impurities like unreacted chloropyridine (retention time ~8.2 minutes) or oxidation products.
- ²⁹Si NMR : Detect silica gel leaching from chromatography columns if present .
Q. How can researchers reconcile discrepancies between computational predictions and experimental reaction yields?
- Solvent/Implicit Models : Re-run DFT calculations with explicit solvent molecules (e.g., water, ethanol) to account for solvation effects.
- Transition State Analysis : Locate energy barriers using Nudged Elastic Band (NEB) methods.
- Experimental Replicates : Conduct ≥3 independent trials to assess statistical significance of yield variations .
Properties
IUPAC Name |
(6-fluoropyridin-2-yl)hydrazine | |
---|---|---|
Source | PubChem | |
URL | https://pubchem.ncbi.nlm.nih.gov | |
Description | Data deposited in or computed by PubChem | |
InChI |
InChI=1S/C5H6FN3/c6-4-2-1-3-5(8-4)9-7/h1-3H,7H2,(H,8,9) | |
Source | PubChem | |
URL | https://pubchem.ncbi.nlm.nih.gov | |
Description | Data deposited in or computed by PubChem | |
InChI Key |
GESCDDOSISTEKA-UHFFFAOYSA-N | |
Source | PubChem | |
URL | https://pubchem.ncbi.nlm.nih.gov | |
Description | Data deposited in or computed by PubChem | |
Canonical SMILES |
C1=CC(=NC(=C1)F)NN | |
Source | PubChem | |
URL | https://pubchem.ncbi.nlm.nih.gov | |
Description | Data deposited in or computed by PubChem | |
Molecular Formula |
C5H6FN3 | |
Source | PubChem | |
URL | https://pubchem.ncbi.nlm.nih.gov | |
Description | Data deposited in or computed by PubChem | |
DSSTOX Substance ID |
DTXSID30363074 | |
Record name | 2-fluoro-6-hydrazinopyridine | |
Source | EPA DSSTox | |
URL | https://comptox.epa.gov/dashboard/DTXSID30363074 | |
Description | DSSTox provides a high quality public chemistry resource for supporting improved predictive toxicology. | |
Molecular Weight |
127.12 g/mol | |
Source | PubChem | |
URL | https://pubchem.ncbi.nlm.nih.gov | |
Description | Data deposited in or computed by PubChem | |
CAS No. |
80714-39-2 | |
Record name | 2-fluoro-6-hydrazinopyridine | |
Source | EPA DSSTox | |
URL | https://comptox.epa.gov/dashboard/DTXSID30363074 | |
Description | DSSTox provides a high quality public chemistry resource for supporting improved predictive toxicology. | |
Synthesis routes and methods
Procedure details
Disclaimer and Information on In-Vitro Research Products
Please be aware that all articles and product information presented on BenchChem are intended solely for informational purposes. The products available for purchase on BenchChem are specifically designed for in-vitro studies, which are conducted outside of living organisms. In-vitro studies, derived from the Latin term "in glass," involve experiments performed in controlled laboratory settings using cells or tissues. It is important to note that these products are not categorized as medicines or drugs, and they have not received approval from the FDA for the prevention, treatment, or cure of any medical condition, ailment, or disease. We must emphasize that any form of bodily introduction of these products into humans or animals is strictly prohibited by law. It is essential to adhere to these guidelines to ensure compliance with legal and ethical standards in research and experimentation.