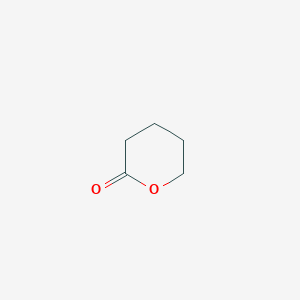
delta-Valerolactone
Overview
Description
Delta-Valerolactone (DVL) is a lactone used as a chemical intermediate in processes such as the production of polyesters . It is a versatile intermediate with a variety of applications, including the manufacturing of coatings and dispersing agents . It is typically used as a co-monomer for polycaprolactone polymers effectively reducing the overall polymer melting point .
Synthesis Analysis
Delta-Valerolactone can be obtained by catalytic processes from nonedible lignocellulose biomass . A highly efficient synthesis of DVL was realized over cheap Cu catalysts through catalytic coupling of 1,5-pentanediol (1,5-PDO) dehydrogenation and ethyl levulinate (EL) hydrogenation . Another method involves the Baeyer–Villiger oxidation of cyclopentanone .Molecular Structure Analysis
Delta-Valerolactone is a cyclic ester . Its molecular formula is C5H8O2 .Chemical Reactions Analysis
Delta-Valerolactone can undergo ring-opening polymerization to form polyesters . It can also undergo chemical transformations, such as esterification and transesterification reactions .Physical And Chemical Properties Analysis
Delta-Valerolactone is a colorless to slightly yellow liquid . It possesses good thermal stability, with a high boiling point and low vapor pressure . It exhibits excellent solvency power, making it a versatile solvent for a wide range of substances, including both polar and non-polar compounds .Scientific Research Applications
Sustainable Energy Production
Delta-Valerolactone plays a significant role in the sustainable production of energy. It can be synthesized from nonedible lignocellulose biomass through catalytic processes . This method is environmentally friendly and cost-effective, making DVL a valuable chemical in the field of sustainable energy.
Biodegradable Polymers
DVL is used in the synthesis of low molecular weight poly(delta-valerolactone) (PVL), which is a biodegradable polymer . This polymer is synthesized through bulk-ring openings polymerization, with boric acid as a catalyst and benzyl alcohol as an initiator. The biodegradability of PVL makes it suitable for medical and environmental applications.
Chemical Intermediate in Polymer Synthesis
As a chemical intermediate, DVL is employed in the synthesis of various polymers . It is particularly useful in creating copolyesters through lipase-catalyzed ring-opening polymerization, which is a method that contributes to the development of new materials with potential applications in packaging, textiles, and biomedical devices.
Ring-Opening Polymerization
DVL serves as a monomer unit in the synthesis of poly(delta-valerolactone)s and poly(conjugated ester)s via ring-opening polymerization . This application is crucial for producing polymers with specific properties for targeted uses in industries such as pharmaceuticals and agriculture.
Catalysis and Chemical Reactions
The compound is involved in catalytic coupling reactions, which are essential for the synchronized production of gamma-valerolactone (GVL) and DVL . These reactions are significant for the chemical industry, as they allow for the efficient production of valuable chemicals without the use of solvents or additional hydrogen.
Mechanism of Action
Target of Action
Delta-Valerolactone (DVL) is primarily used as a chemical intermediate in the manufacturing of coatings and dispersing agents . It is typically used as a co-monomer for polycaprolactone polymers, effectively reducing the overall polymer melting point .
Mode of Action
The mode of action of DVL involves the process of ring-opening polymerization (ROP). When employed in the ROP of DVL in combination with 7-methyl-1,5,7-triazabicyclo[4.4.0]dec-5-ene (MTBD), a fast and “living”/controlled feature was observed in both solvent-free polymerization and solution polymerization . This process was proved by kinetic and chain extension experiments .
Biochemical Pathways
The biochemical pathway of DVL involves the ring-opening polymerization (ROP) of lactones . This is a highly efficient method for synthesizing aliphatic polyester materials . A novel series of benzoheterocyclic urea catalysts has been synthesized and characterized for this purpose .
Pharmacokinetics
It’s known that dvl is used in the synthesis of an acid chloride, which is subsequently utilized as a building block in active ingredients .
Result of Action
The result of DVL’s action is the production of polyvalerolactones (PVLs) with different molecular weights and narrow molecular weight distributions . The PVLs with different topological structures were prepared by varying the initiators . The structure and thermal properties of the resulting polymers were determined by NMR, GPC, and DSC analyses .
Action Environment
The action environment of DVL can influence its action, efficacy, and stability. For instance, the large energy released from ethyl levulinate (EL) hydrogenation can be utilized effectively for endothermic 1,5-PDO dehydrogenation . Moreover, DVL is known for its low toxicity and favorable environmental profile. It is biodegradable under aerobic conditions, reducing its impact on the environment . Its biocompatibility makes it suitable for use in pharmaceutical and biomedical applications .
Safety and Hazards
Future Directions
Delta-Valerolactone shows promise in diverse fields such as pharmaceuticals, materials science, and organic synthesis . It has been explored in drug-carrier systems, showing promise as an effective carrier for oral administration of drugs . It has also been utilized in the preparation of functional polyesters for various industrial applications .
properties
IUPAC Name |
oxan-2-one | |
---|---|---|
Source | PubChem | |
URL | https://pubchem.ncbi.nlm.nih.gov | |
Description | Data deposited in or computed by PubChem | |
InChI |
InChI=1S/C5H8O2/c6-5-3-1-2-4-7-5/h1-4H2 | |
Source | PubChem | |
URL | https://pubchem.ncbi.nlm.nih.gov | |
Description | Data deposited in or computed by PubChem | |
InChI Key |
OZJPLYNZGCXSJM-UHFFFAOYSA-N | |
Source | PubChem | |
URL | https://pubchem.ncbi.nlm.nih.gov | |
Description | Data deposited in or computed by PubChem | |
Canonical SMILES |
C1CCOC(=O)C1 | |
Source | PubChem | |
URL | https://pubchem.ncbi.nlm.nih.gov | |
Description | Data deposited in or computed by PubChem | |
Molecular Formula |
C5H8O2 | |
Source | PubChem | |
URL | https://pubchem.ncbi.nlm.nih.gov | |
Description | Data deposited in or computed by PubChem | |
Related CAS |
26354-94-9 | |
Record name | Poly-δ-valerolactone | |
Source | CAS Common Chemistry | |
URL | https://commonchemistry.cas.org/detail?cas_rn=26354-94-9 | |
Description | CAS Common Chemistry is an open community resource for accessing chemical information. Nearly 500,000 chemical substances from CAS REGISTRY cover areas of community interest, including common and frequently regulated chemicals, and those relevant to high school and undergraduate chemistry classes. This chemical information, curated by our expert scientists, is provided in alignment with our mission as a division of the American Chemical Society. | |
Explanation | The data from CAS Common Chemistry is provided under a CC-BY-NC 4.0 license, unless otherwise stated. | |
DSSTOX Substance ID |
DTXSID6044438 | |
Record name | Tetrahydro-2H-pyran-2-one | |
Source | EPA DSSTox | |
URL | https://comptox.epa.gov/dashboard/DTXSID6044438 | |
Description | DSSTox provides a high quality public chemistry resource for supporting improved predictive toxicology. | |
Molecular Weight |
100.12 g/mol | |
Source | PubChem | |
URL | https://pubchem.ncbi.nlm.nih.gov | |
Description | Data deposited in or computed by PubChem | |
Physical Description |
Liquid | |
Record name | 2H-Pyran-2-one, tetrahydro- | |
Source | EPA Chemicals under the TSCA | |
URL | https://www.epa.gov/chemicals-under-tsca | |
Description | EPA Chemicals under the Toxic Substances Control Act (TSCA) collection contains information on chemicals and their regulations under TSCA, including non-confidential content from the TSCA Chemical Substance Inventory and Chemical Data Reporting. | |
Product Name |
delta-Valerolactone | |
CAS RN |
542-28-9, 26354-94-9 | |
Record name | δ-Valerolactone | |
Source | CAS Common Chemistry | |
URL | https://commonchemistry.cas.org/detail?cas_rn=542-28-9 | |
Description | CAS Common Chemistry is an open community resource for accessing chemical information. Nearly 500,000 chemical substances from CAS REGISTRY cover areas of community interest, including common and frequently regulated chemicals, and those relevant to high school and undergraduate chemistry classes. This chemical information, curated by our expert scientists, is provided in alignment with our mission as a division of the American Chemical Society. | |
Explanation | The data from CAS Common Chemistry is provided under a CC-BY-NC 4.0 license, unless otherwise stated. | |
Record name | delta-Valerolactone | |
Source | ChemIDplus | |
URL | https://pubchem.ncbi.nlm.nih.gov/substance/?source=chemidplus&sourceid=0000542289 | |
Description | ChemIDplus is a free, web search system that provides access to the structure and nomenclature authority files used for the identification of chemical substances cited in National Library of Medicine (NLM) databases, including the TOXNET system. | |
Record name | Poly-delta-valerolactone | |
Source | ChemIDplus | |
URL | https://pubchem.ncbi.nlm.nih.gov/substance/?source=chemidplus&sourceid=0026354949 | |
Description | ChemIDplus is a free, web search system that provides access to the structure and nomenclature authority files used for the identification of chemical substances cited in National Library of Medicine (NLM) databases, including the TOXNET system. | |
Record name | NSC65442 | |
Source | DTP/NCI | |
URL | https://dtp.cancer.gov/dtpstandard/servlet/dwindex?searchtype=NSC&outputformat=html&searchlist=65442 | |
Description | The NCI Development Therapeutics Program (DTP) provides services and resources to the academic and private-sector research communities worldwide to facilitate the discovery and development of new cancer therapeutic agents. | |
Explanation | Unless otherwise indicated, all text within NCI products is free of copyright and may be reused without our permission. Credit the National Cancer Institute as the source. | |
Record name | 5-Valerolactone | |
Source | DTP/NCI | |
URL | https://dtp.cancer.gov/dtpstandard/servlet/dwindex?searchtype=NSC&outputformat=html&searchlist=6247 | |
Description | The NCI Development Therapeutics Program (DTP) provides services and resources to the academic and private-sector research communities worldwide to facilitate the discovery and development of new cancer therapeutic agents. | |
Explanation | Unless otherwise indicated, all text within NCI products is free of copyright and may be reused without our permission. Credit the National Cancer Institute as the source. | |
Record name | 2H-Pyran-2-one, tetrahydro- | |
Source | EPA Chemicals under the TSCA | |
URL | https://www.epa.gov/chemicals-under-tsca | |
Description | EPA Chemicals under the Toxic Substances Control Act (TSCA) collection contains information on chemicals and their regulations under TSCA, including non-confidential content from the TSCA Chemical Substance Inventory and Chemical Data Reporting. | |
Record name | Tetrahydro-2H-pyran-2-one | |
Source | EPA DSSTox | |
URL | https://comptox.epa.gov/dashboard/DTXSID6044438 | |
Description | DSSTox provides a high quality public chemistry resource for supporting improved predictive toxicology. | |
Record name | δ-valerolactone | |
Source | European Chemicals Agency (ECHA) | |
URL | https://echa.europa.eu/substance-information/-/substanceinfo/100.008.007 | |
Description | The European Chemicals Agency (ECHA) is an agency of the European Union which is the driving force among regulatory authorities in implementing the EU's groundbreaking chemicals legislation for the benefit of human health and the environment as well as for innovation and competitiveness. | |
Explanation | Use of the information, documents and data from the ECHA website is subject to the terms and conditions of this Legal Notice, and subject to other binding limitations provided for under applicable law, the information, documents and data made available on the ECHA website may be reproduced, distributed and/or used, totally or in part, for non-commercial purposes provided that ECHA is acknowledged as the source: "Source: European Chemicals Agency, http://echa.europa.eu/". Such acknowledgement must be included in each copy of the material. ECHA permits and encourages organisations and individuals to create links to the ECHA website under the following cumulative conditions: Links can only be made to webpages that provide a link to the Legal Notice page. | |
Record name | DELTA-VALEROLACTONE | |
Source | FDA Global Substance Registration System (GSRS) | |
URL | https://gsrs.ncats.nih.gov/ginas/app/beta/substances/14V1X9149L | |
Description | The FDA Global Substance Registration System (GSRS) enables the efficient and accurate exchange of information on what substances are in regulated products. Instead of relying on names, which vary across regulatory domains, countries, and regions, the GSRS knowledge base makes it possible for substances to be defined by standardized, scientific descriptions. | |
Explanation | Unless otherwise noted, the contents of the FDA website (www.fda.gov), both text and graphics, are not copyrighted. They are in the public domain and may be republished, reprinted and otherwise used freely by anyone without the need to obtain permission from FDA. Credit to the U.S. Food and Drug Administration as the source is appreciated but not required. | |
Synthesis routes and methods
Procedure details
Retrosynthesis Analysis
AI-Powered Synthesis Planning: Our tool employs the Template_relevance Pistachio, Template_relevance Bkms_metabolic, Template_relevance Pistachio_ringbreaker, Template_relevance Reaxys, Template_relevance Reaxys_biocatalysis model, leveraging a vast database of chemical reactions to predict feasible synthetic routes.
One-Step Synthesis Focus: Specifically designed for one-step synthesis, it provides concise and direct routes for your target compounds, streamlining the synthesis process.
Accurate Predictions: Utilizing the extensive PISTACHIO, BKMS_METABOLIC, PISTACHIO_RINGBREAKER, REAXYS, REAXYS_BIOCATALYSIS database, our tool offers high-accuracy predictions, reflecting the latest in chemical research and data.
Strategy Settings
Precursor scoring | Relevance Heuristic |
---|---|
Min. plausibility | 0.01 |
Model | Template_relevance |
Template Set | Pistachio/Bkms_metabolic/Pistachio_ringbreaker/Reaxys/Reaxys_biocatalysis |
Top-N result to add to graph | 6 |
Feasible Synthetic Routes
Q & A
Q1: What is the molecular formula and weight of δ-valerolactone?
A1: δ-Valerolactone has a molecular formula of C5H8O2 and a molecular weight of 100.12 g/mol.
Q2: What makes δ-valerolactone prone to polymerization, while γ-butyrolactone does not, despite both having ring strain?
A2: Although both lactones possess ring strain, the key difference lies in the degree of strain and conformational preferences. δ-Valerolactone experiences greater geometric distortion in its ester group compared to γ-butyrolactone []. Additionally, model compounds of poly-4-hydroxybutyrate, the polymer of γ-butyrolactone, demonstrate significant stability in coiled conformations, further hindering polymerization [, ].
Q3: How does the ring strain of δ-valerolactone compare to other lactones like ε-caprolactone and 1,4-dioxan-2-one?
A3: Theoretical investigations reveal that the ring strain in δ-valerolactone is higher than in ε-caprolactone and 1,4-dioxan-2-one, but lower than in γ-butyrolactone []. This correlates with the exergonic character of the ring-opening process, suggesting that the higher the strain, the more favorable the polymerization [].
Q4: How does the environment influence the polymerizability of δ-valerolactone?
A4: Environmental factors, particularly solvent polarity, significantly affect the polymerizability of δ-valerolactone. Polar solvents stabilize the monomeric form, while less polar environments favor polymerization. This highlights the importance of considering solvent effects in reactions involving this lactone [].
Q5: Can you describe the palladium-catalyzed decarboxylative [4+3] cyclization reaction of δ-valerolactone with 1,1-dicyanocyclopropanes?
A5: This reaction utilizes a palladium catalyst to facilitate the formation of cycloheptane derivatives from γ-methylidene-δ-valerolactones and 1,1-dicyanocyclopropanes []. Notably, this methodology exhibits versatility, enabling the synthesis of azepanes through reactions with aziridines, including their asymmetric variants [].
Q6: How does changing the electronic properties of triarylphosphine ligands impact the palladium-catalyzed decarboxylative cyclization of δ-valerolactone with isocyanates?
A6: The product distribution between piperidones and azaspiro[2.4]heptanones in this reaction is strongly influenced by the electronic properties of the triarylphosphine ligands []. Electron-rich ligands favor the formation of azaspiro[2.4]heptanones, highlighting the ability to tune product selectivity through ligand modification [].
Q7: Can you elaborate on the use of δ-valerolactone in synthesizing nipecotic acid derivatives via palladium-catalyzed reactions?
A7: This reaction employs a palladium catalyst to enable the decarboxylative cyclization of γ-methylidene-δ-valerolactones with imines, yielding multisubstituted nipecotic acid (piperidine-3-carboxylic acid) derivatives []. Employing a diethoxyphosphinoyl group as the N-protecting group on the imine ensures high diastereoselectivity, and the resulting products are amenable to further derivatization [].
Q8: How is δ-valerolactone used in preparing biodegradable amphiphilic block copolymers?
A8: δ-Valerolactone is a valuable monomer for synthesizing biodegradable block copolymers like methoxy poly(ethylene glycol)-block-poly(δ-valerolactone). These copolymers can self-assemble into micelles in aqueous solutions, making them promising candidates for drug delivery systems [, , ].
Q9: What is the impact of incorporating δ-valerolactone into polycaprolactone-based antifouling coatings?
A9: Incorporating δ-valerolactone into polycaprolactone-based coatings disrupts the polymer's crystallinity, leading to faster degradation and controlled release of bioactive molecules []. This property is beneficial in antifouling applications, where a controlled release of biocides is desired over time.
Q10: What role does δ-valerolactone play in the development of pasty-type implantable drug delivery devices?
A10: δ-Valerolactone, when copolymerized with ε-caprolactone, forms biocompatible and biodegradable pasty-type copolyesters suitable for implantable drug delivery devices []. The degradation rate of these devices can be modulated by the copolymer composition and the hydrophilicity of the encapsulated drug [].
Q11: What analytical techniques are commonly used to characterize and quantify δ-valerolactone and its derivatives?
A11: Common techniques include:
- Nuclear Magnetic Resonance (NMR) Spectroscopy: Structural elucidation and compositional analysis of δ-valerolactone-based polymers [, , ].
- Gel Permeation Chromatography (GPC): Determination of molecular weight and molecular weight distribution of polymers containing δ-valerolactone [, ].
- Differential Scanning Calorimetry (DSC): Thermal analysis of δ-valerolactone-based polymers, including melting temperature and glass transition temperature [, , ].
- Gas Chromatography-Mass Spectrometry (GC-MS): Analysis of δ-valerolactone and its metabolites in biological samples [].
Q12: What are the challenges associated with the analytical detection of δ-valerolactone in biological matrices, and how are they addressed?
A12: The presence of salts, particularly the sodium salt of δ-valerolactone, poses a challenge for GC-MS analysis due to its non-volatility. This necessitates derivatization prior to analysis. N-methyl-N-(t-butyldimethylsilyl)trifluoroacetamide (MTBSTFA) has proven effective for this purpose, preventing intramolecular cyclization during derivatization [].
Q13: How have computational methods been applied to study the conformational preferences of δ-valerolactone and its polymer?
A13: Ab initio calculations have been instrumental in understanding the conformational flexibility of δ-valerolactone []. Calculations revealed that various conformations, including half-chair, boat, chair, envelope, and skew, are accessible within a relatively low energy range, highlighting the molecule's conformational diversity [].
Q14: How do structural modifications to γ-methylidene-δ-valerolactones impact the diastereoselectivity of palladium-catalyzed decarboxylative lactamization with isocyanates?
A14: The enantioselectivity of this reaction can be significantly influenced by modifying both the ester group on the lactone and the substituents on the phosphoramidite ligand []. Optimizing these structural features allows for fine-tuning the reaction to achieve high levels of enantiomeric excess in the desired lactam product [].
Disclaimer and Information on In-Vitro Research Products
Please be aware that all articles and product information presented on BenchChem are intended solely for informational purposes. The products available for purchase on BenchChem are specifically designed for in-vitro studies, which are conducted outside of living organisms. In-vitro studies, derived from the Latin term "in glass," involve experiments performed in controlled laboratory settings using cells or tissues. It is important to note that these products are not categorized as medicines or drugs, and they have not received approval from the FDA for the prevention, treatment, or cure of any medical condition, ailment, or disease. We must emphasize that any form of bodily introduction of these products into humans or animals is strictly prohibited by law. It is essential to adhere to these guidelines to ensure compliance with legal and ethical standards in research and experimentation.