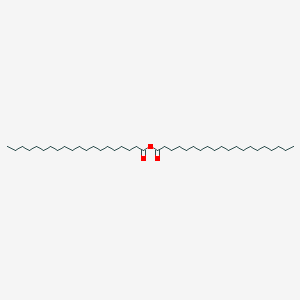
Arachidic anhydride
Overview
Description
Arachidic anhydride, also known as eicosanoic anhydride, is a chemical compound with the molecular formula C40H78O3 and a molecular weight of 607.05 g/mol . It is derived from arachidic acid (eicosanoic acid), a saturated fatty acid commonly found in peanut oil and other vegetable oils. This compound is primarily used in organic synthesis and industrial applications due to its reactivity and ability to form various derivatives.
Mechanism of Action
Target of Action
Arachidic anhydride, also known as eicosanoic anhydride , is a derivative of arachidonic acid. Arachidonic acid is known to interact with several targets, including the Peroxisome proliferator-activated receptor alpha , Bile acid receptor , Retinoic acid receptor RXR-alpha , Prostaglandin G/H synthase 1 , and the 14 kDa fatty acid-binding protein . These targets play crucial roles in various physiological processes, such as cell repair and growth, inflammation, and the biosynthesis of prostaglandins, thromboxanes, and leukotrienes .
Mode of Action
Arachidonic acid, from which this compound is derived, is metabolized by cyclooxygenases (COXs) , lipoxygenases (LOXs) , and cytochrome P450 (CYP) enzymes . These enzymes convert arachidonic acid into a spectrum of bioactive mediators, including prostanoids, leukotrienes, epoxyeicosatrienoic acids (EETs), dihydroxyeicosatetraenoic acid (diHETEs), eicosatetraenoic acids (ETEs), and lipoxins . These mediators are involved in various physiological and
Preparation Methods
Synthetic Routes and Reaction Conditions: Arachidic anhydride can be synthesized through the nucleophilic acyl substitution reaction of an acid chloride with a carboxylate anion . The general reaction involves the following steps:
- Preparation of arachidic acid chloride by reacting arachidic acid with thionyl chloride (SOCl2) or oxalyl chloride (COCl)2.
- Reaction of the resulting arachidic acid chloride with the sodium salt of arachidic acid to form this compound.
Industrial Production Methods: Industrial production of this compound typically involves the same synthetic route but on a larger scale. The reaction conditions are optimized to ensure high yield and purity. The process may involve the use of solvents such as pyridine to facilitate the reaction and control the temperature to avoid side reactions.
Chemical Reactions Analysis
Types of Reactions: Arachidic anhydride undergoes several types of chemical reactions, including:
Hydrolysis: Reacts with water to form arachidic acid.
Alcoholysis: Reacts with alcohols to form esters.
Aminolysis: Reacts with amines to form amides.
Reduction: Can be reduced to primary alcohols using reducing agents like lithium aluminum hydride (LiAlH4).
Common Reagents and Conditions:
Hydrolysis: Water, often with a catalytic amount of acid or base.
Alcoholysis: Alcohols such as methanol or ethanol, often with a base catalyst.
Aminolysis: Amines such as ammonia or primary amines, often with a base catalyst.
Reduction: Lithium aluminum hydride (LiAlH4) in anhydrous ether.
Major Products Formed:
Hydrolysis: Arachidic acid.
Alcoholysis: Arachidic esters.
Aminolysis: Arachidic amides.
Reduction: Arachidyl alcohol.
Scientific Research Applications
Arachidic anhydride has several applications in scientific research, including:
Organic Synthesis: Used as a reagent in the synthesis of various organic compounds, including pharmaceuticals and agrochemicals.
Polymer Chemistry: Utilized in the preparation of polymers and copolymers with specific properties.
Biological Studies: Employed in the study of fatty acid metabolism and the synthesis of biologically active molecules.
Industrial Applications: Used in the production of surfactants, lubricants, and plasticizers.
Comparison with Similar Compounds
- Acetic Anhydride
- Succinic Anhydride
- Phthalic Anhydride
- Maleic Anhydride
Properties
IUPAC Name |
icosanoyl icosanoate | |
---|---|---|
Source | PubChem | |
URL | https://pubchem.ncbi.nlm.nih.gov | |
Description | Data deposited in or computed by PubChem | |
InChI |
InChI=1S/C40H78O3/c1-3-5-7-9-11-13-15-17-19-21-23-25-27-29-31-33-35-37-39(41)43-40(42)38-36-34-32-30-28-26-24-22-20-18-16-14-12-10-8-6-4-2/h3-38H2,1-2H3 | |
Source | PubChem | |
URL | https://pubchem.ncbi.nlm.nih.gov | |
Description | Data deposited in or computed by PubChem | |
InChI Key |
AUONNNVJUCSETH-UHFFFAOYSA-N | |
Source | PubChem | |
URL | https://pubchem.ncbi.nlm.nih.gov | |
Description | Data deposited in or computed by PubChem | |
Canonical SMILES |
CCCCCCCCCCCCCCCCCCCC(=O)OC(=O)CCCCCCCCCCCCCCCCCCC | |
Source | PubChem | |
URL | https://pubchem.ncbi.nlm.nih.gov | |
Description | Data deposited in or computed by PubChem | |
Molecular Formula |
C40H78O3 | |
Source | PubChem | |
URL | https://pubchem.ncbi.nlm.nih.gov | |
Description | Data deposited in or computed by PubChem | |
DSSTOX Substance ID |
DTXSID20340753 | |
Record name | Arachidic anhydride | |
Source | EPA DSSTox | |
URL | https://comptox.epa.gov/dashboard/DTXSID20340753 | |
Description | DSSTox provides a high quality public chemistry resource for supporting improved predictive toxicology. | |
Molecular Weight |
607.0 g/mol | |
Source | PubChem | |
URL | https://pubchem.ncbi.nlm.nih.gov | |
Description | Data deposited in or computed by PubChem | |
CAS No. |
55726-22-2 | |
Record name | Eicosanoic acid, 1,1′-anhydride | |
Source | CAS Common Chemistry | |
URL | https://commonchemistry.cas.org/detail?cas_rn=55726-22-2 | |
Description | CAS Common Chemistry is an open community resource for accessing chemical information. Nearly 500,000 chemical substances from CAS REGISTRY cover areas of community interest, including common and frequently regulated chemicals, and those relevant to high school and undergraduate chemistry classes. This chemical information, curated by our expert scientists, is provided in alignment with our mission as a division of the American Chemical Society. | |
Explanation | The data from CAS Common Chemistry is provided under a CC-BY-NC 4.0 license, unless otherwise stated. | |
Record name | Arachidic anhydride | |
Source | EPA DSSTox | |
URL | https://comptox.epa.gov/dashboard/DTXSID20340753 | |
Description | DSSTox provides a high quality public chemistry resource for supporting improved predictive toxicology. | |
Record name | Arachidic Anhydride | |
Source | European Chemicals Agency (ECHA) | |
URL | https://echa.europa.eu/information-on-chemicals | |
Description | The European Chemicals Agency (ECHA) is an agency of the European Union which is the driving force among regulatory authorities in implementing the EU's groundbreaking chemicals legislation for the benefit of human health and the environment as well as for innovation and competitiveness. | |
Explanation | Use of the information, documents and data from the ECHA website is subject to the terms and conditions of this Legal Notice, and subject to other binding limitations provided for under applicable law, the information, documents and data made available on the ECHA website may be reproduced, distributed and/or used, totally or in part, for non-commercial purposes provided that ECHA is acknowledged as the source: "Source: European Chemicals Agency, http://echa.europa.eu/". Such acknowledgement must be included in each copy of the material. ECHA permits and encourages organisations and individuals to create links to the ECHA website under the following cumulative conditions: Links can only be made to webpages that provide a link to the Legal Notice page. | |
Retrosynthesis Analysis
AI-Powered Synthesis Planning: Our tool employs the Template_relevance Pistachio, Template_relevance Bkms_metabolic, Template_relevance Pistachio_ringbreaker, Template_relevance Reaxys, Template_relevance Reaxys_biocatalysis model, leveraging a vast database of chemical reactions to predict feasible synthetic routes.
One-Step Synthesis Focus: Specifically designed for one-step synthesis, it provides concise and direct routes for your target compounds, streamlining the synthesis process.
Accurate Predictions: Utilizing the extensive PISTACHIO, BKMS_METABOLIC, PISTACHIO_RINGBREAKER, REAXYS, REAXYS_BIOCATALYSIS database, our tool offers high-accuracy predictions, reflecting the latest in chemical research and data.
Strategy Settings
Precursor scoring | Relevance Heuristic |
---|---|
Min. plausibility | 0.01 |
Model | Template_relevance |
Template Set | Pistachio/Bkms_metabolic/Pistachio_ringbreaker/Reaxys/Reaxys_biocatalysis |
Top-N result to add to graph | 6 |
Feasible Synthetic Routes
Q1: How does arachidic anhydride interact with graphite to form chiral structures despite being an achiral molecule?
A1: this compound, with its two identical alkyl chains connected by an anhydride group, adopts an extended conformation upon adsorption onto graphite. [] This extended form exhibits a shift between the alkyl chains along the molecular backbone. Through weak van der Waals forces, the molecules self-assemble into highly ordered, two-dimensional domains on the graphite surface. Interestingly, this self-assembly process spontaneously generates two types of domains (labeled 'm' and 'm'') that are mirror images of each other. These domains, though formed from an achiral molecule, exhibit opposite chiralities due to the specific arrangement of the alkyl chains relative to the row-packing direction within each domain. This phenomenon highlights the ability of even simple, achiral molecules to form complex, chiral structures through self-assembly.
Disclaimer and Information on In-Vitro Research Products
Please be aware that all articles and product information presented on BenchChem are intended solely for informational purposes. The products available for purchase on BenchChem are specifically designed for in-vitro studies, which are conducted outside of living organisms. In-vitro studies, derived from the Latin term "in glass," involve experiments performed in controlled laboratory settings using cells or tissues. It is important to note that these products are not categorized as medicines or drugs, and they have not received approval from the FDA for the prevention, treatment, or cure of any medical condition, ailment, or disease. We must emphasize that any form of bodily introduction of these products into humans or animals is strictly prohibited by law. It is essential to adhere to these guidelines to ensure compliance with legal and ethical standards in research and experimentation.