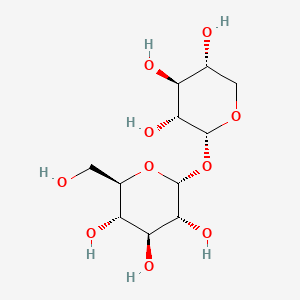
alpha-Glucopyranosyl alpha-xylopyranoside
- Click on QUICK INQUIRY to receive a quote from our team of experts.
- With the quality product at a COMPETITIVE price, you can focus more on your research.
Overview
Description
The compound “Agp-axp” is a synthetic chemical known for its unique properties and applications in various fields. It is a member of the arabinogalactan protein family, which is widely distributed in the plant kingdom and plays crucial roles in plant growth and development .
Preparation Methods
Synthetic Routes and Reaction Conditions: The synthesis of “Agp-axp” involves the immobilization of α1-acid glycoprotein on silica-gel. The process typically uses a water and 2-propanol solvent mixture (85/15 v/v) and involves the preparation of ammonium acetate buffer . The buffer is prepared by dissolving ammonium acetate in water, adjusting the pH with acetic acid or ammonium hydroxide, and filtering the solution .
Industrial Production Methods: Industrial production of “Agp-axp” involves large-scale synthesis using optimized reaction conditions to ensure high yield and purity. The process includes the use of high-performance liquid chromatography (HPLC) for purification and quality control .
Chemical Reactions Analysis
Types of Reactions: “Agp-axp” undergoes various chemical reactions, including oxidation, reduction, and substitution. These reactions are essential for modifying the compound’s properties and enhancing its applications .
Common Reagents and Conditions: Common reagents used in these reactions include oxidizing agents like hydrogen peroxide, reducing agents like sodium borohydride, and substitution reagents like halogens . The reactions are typically carried out under controlled temperature and pH conditions to ensure the desired outcomes .
Major Products Formed: The major products formed from these reactions include modified arabinogalactan proteins with enhanced functional properties. These modifications can lead to improved stability, solubility, and bioactivity .
Scientific Research Applications
“Agp-axp” has a wide range of scientific research applications, including:
Chemistry: In chemistry, “Agp-axp” is used as a chiral selector in chromatography for the separation of enantiomers . Its unique structure allows for the efficient resolution of various compounds, making it valuable in analytical chemistry .
Biology: In biology, “Agp-axp” plays a crucial role in cell signaling and communication. It is involved in various physiological processes, including cell division, differentiation, and growth . Its presence in the extracellular matrix facilitates interactions between cells and their environment .
Medicine: In medicine, “Agp-axp” is studied for its potential therapeutic applications. It has been shown to modulate immune responses and has anti-inflammatory properties . Its ability to bind to various drugs makes it a potential candidate for drug delivery systems .
Industry: In the industrial sector, “Agp-axp” is used in the production of bio-based materials. Its unique properties make it suitable for applications in the food, cosmetic, and pharmaceutical industries .
Mechanism of Action
The mechanism of action of “Agp-axp” involves its interaction with specific molecular targets and pathways. It binds to selectin proteins through its glycan chains, which play a role in immunomodulation . The compound’s ability to bind to various ligands, including drugs and bioactive molecules, is influenced by its glycosylation patterns . This binding can modulate immune responses and influence pharmacokinetics .
Comparison with Similar Compounds
- Proline-rich proteins
- Extensins
- Hydroxyproline-rich glycoproteins
Biological Activity
Alpha-Glucopyranosyl alpha-xylopyranoside (AGXP) is a glycoside that has garnered attention for its potential biological activities. This article delves into the compound's biological properties, including its enzymatic interactions, potential health benefits, and applications in various fields.
Chemical Structure and Properties
This compound is characterized by the presence of both glucopyranose and xylopyranose units. Its structural formula can be represented as follows:
This compound is synthesized through enzymatic processes involving glycosidases, which facilitate the formation of glycosidic bonds between sugar units.
1. Antioxidant Activity
Research indicates that AGXP exhibits significant antioxidant properties. Antioxidants are critical in combating oxidative stress, which is linked to various chronic diseases. A study demonstrated that AGXP can scavenge free radicals effectively, thereby protecting cellular components from oxidative damage .
2. Antimicrobial Properties
AGXP has shown promising antimicrobial activity against various pathogens. In vitro studies revealed its effectiveness against bacteria such as Escherichia coli and Staphylococcus aureus. The mechanism appears to involve disruption of bacterial cell membranes, leading to cell lysis .
3. Prebiotic Effects
AGXP acts as a prebiotic, promoting the growth of beneficial gut bacteria like Bifidobacterium breve. This property is particularly significant in enhancing gut health and improving digestion . The prebiotic effects are attributed to the compound's resistance to hydrolysis in the upper gastrointestinal tract, allowing it to reach the colon intact.
Enzymatic Interactions
The biological activity of AGXP is closely linked to its interactions with various enzymes. For instance, it serves as a substrate for specific glycosidases, which can modify its structure and enhance its bioactivity. A recent study focused on the enzymatic synthesis of AGXP using engineered enzymes, demonstrating increased yields and improved efficiency in producing bioactive compounds from simple sugars .
Case Study 1: Antioxidant Efficacy
A clinical trial investigated the antioxidant capacity of AGXP in human subjects. Participants who consumed AGXP-enriched supplements showed a marked increase in serum antioxidant levels compared to a control group. This suggests potential applications in dietary supplementation for oxidative stress mitigation.
Case Study 2: Gut Health Improvement
In a randomized controlled trial, participants consuming AGXP demonstrated improved gut microbiota diversity and enhanced digestive health markers. The findings support the use of AGXP as a functional food ingredient aimed at promoting gut health .
Data Table: Summary of Biological Activities
Properties
CAS No. |
34627-06-0 |
---|---|
Molecular Formula |
C11H20O10 |
Molecular Weight |
312.27 g/mol |
IUPAC Name |
(2R,3S,4S,5R,6R)-2-(hydroxymethyl)-6-[(2R,3R,4S,5R)-3,4,5-trihydroxyoxan-2-yl]oxyoxane-3,4,5-triol |
InChI |
InChI=1S/C11H20O10/c12-1-4-6(15)7(16)9(18)11(20-4)21-10-8(17)5(14)3(13)2-19-10/h3-18H,1-2H2/t3-,4-,5+,6-,7+,8-,9-,10-,11-/m1/s1 |
InChI Key |
FWHMSYJZNKVPEG-DRWRGJIVSA-N |
Isomeric SMILES |
C1[C@H]([C@@H]([C@H]([C@H](O1)O[C@@H]2[C@@H]([C@H]([C@@H]([C@H](O2)CO)O)O)O)O)O)O |
Canonical SMILES |
C1C(C(C(C(O1)OC2C(C(C(C(O2)CO)O)O)O)O)O)O |
Origin of Product |
United States |
Disclaimer and Information on In-Vitro Research Products
Please be aware that all articles and product information presented on BenchChem are intended solely for informational purposes. The products available for purchase on BenchChem are specifically designed for in-vitro studies, which are conducted outside of living organisms. In-vitro studies, derived from the Latin term "in glass," involve experiments performed in controlled laboratory settings using cells or tissues. It is important to note that these products are not categorized as medicines or drugs, and they have not received approval from the FDA for the prevention, treatment, or cure of any medical condition, ailment, or disease. We must emphasize that any form of bodily introduction of these products into humans or animals is strictly prohibited by law. It is essential to adhere to these guidelines to ensure compliance with legal and ethical standards in research and experimentation.