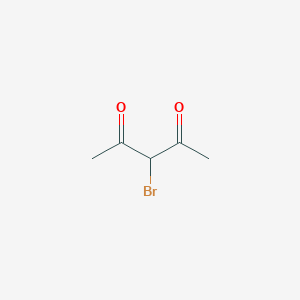
3-Bromopentane-2,4-dione
Overview
Description
3-Bromopentane-2,4-dione is an organic compound with the chemical formula C5H7BrO2. It is a colorless to pale yellow crystalline solid with a weak aroma. This compound is soluble in alcohol, ether, and ketone solvents at room temperature but insoluble in water . It is commonly used as a reagent and intermediate in organic synthesis .
Scientific Research Applications
3-Bromopentane-2,4-dione has a wide range of applications in scientific research:
Organic Synthesis: It is used as a building block in the synthesis of various organic compounds, including pharmaceuticals and agrochemicals.
Medicinal Chemistry: The compound is used in the development of new drugs and therapeutic agents.
Material Science: It is utilized in the synthesis of materials with specific properties for industrial applications.
Safety and Hazards
Future Directions
Preparation Methods
3-Bromopentane-2,4-dione can be synthesized through several methods. One common method involves the bromination of acetylacetone (2,4-pentanedione) using bromine or a brominating agent such as monobromomalononitrile (MBM) . The reaction typically occurs under mild conditions and yields high amounts of the desired product . Another method involves the reaction of 3-bromopentane with sodium acetate in acetic anhydride, followed by an oxidation reaction to convert the intermediate to this compound .
Chemical Reactions Analysis
3-Bromopentane-2,4-dione undergoes various chemical reactions, including:
Substitution Reactions: It can participate in nucleophilic substitution reactions where the bromine atom is replaced by other nucleophiles.
Oxidation Reactions: The compound can be oxidized to form different products depending on the oxidizing agent and conditions used.
Reduction Reactions: Reduction of this compound can lead to the formation of corresponding alcohols or other reduced products.
Common reagents used in these reactions include bromine, sodium acetate, and various oxidizing and reducing agents . The major products formed from these reactions depend on the specific conditions and reagents used.
Mechanism of Action
The mechanism of action of 3-Bromopentane-2,4-dione involves its reactivity as a brominated compound. The bromine atom in the molecule can participate in various chemical reactions, making it a versatile intermediate in organic synthesis . The molecular targets and pathways involved depend on the specific reactions and applications in which the compound is used.
Comparison with Similar Compounds
3-Bromopentane-2,4-dione can be compared with other brominated compounds and 1,3-dicarbonyl compounds. Similar compounds include:
3-Bromo-2,4-pentanedione: Another brominated 1,3-dicarbonyl compound with similar reactivity.
Monobromomalononitrile: A brominating agent used in similar synthetic applications.
The uniqueness of this compound lies in its specific reactivity and applications in organic synthesis, medicinal chemistry, and material science .
Properties
IUPAC Name |
3-bromopentane-2,4-dione | |
---|---|---|
Source | PubChem | |
URL | https://pubchem.ncbi.nlm.nih.gov | |
Description | Data deposited in or computed by PubChem | |
InChI |
InChI=1S/C5H7BrO2/c1-3(7)5(6)4(2)8/h5H,1-2H3 | |
Source | PubChem | |
URL | https://pubchem.ncbi.nlm.nih.gov | |
Description | Data deposited in or computed by PubChem | |
InChI Key |
KCHDFLHQXZRDKL-UHFFFAOYSA-N | |
Source | PubChem | |
URL | https://pubchem.ncbi.nlm.nih.gov | |
Description | Data deposited in or computed by PubChem | |
Canonical SMILES |
CC(=O)C(C(=O)C)Br | |
Source | PubChem | |
URL | https://pubchem.ncbi.nlm.nih.gov | |
Description | Data deposited in or computed by PubChem | |
Molecular Formula |
C5H7BrO2 | |
Source | PubChem | |
URL | https://pubchem.ncbi.nlm.nih.gov | |
Description | Data deposited in or computed by PubChem | |
DSSTOX Substance ID |
DTXSID60326692 | |
Record name | 3-bromopentane-2,4-dione | |
Source | EPA DSSTox | |
URL | https://comptox.epa.gov/dashboard/DTXSID60326692 | |
Description | DSSTox provides a high quality public chemistry resource for supporting improved predictive toxicology. | |
Molecular Weight |
179.01 g/mol | |
Source | PubChem | |
URL | https://pubchem.ncbi.nlm.nih.gov | |
Description | Data deposited in or computed by PubChem | |
CAS No. |
3043-28-5 | |
Record name | 3-bromopentane-2,4-dione | |
Source | EPA DSSTox | |
URL | https://comptox.epa.gov/dashboard/DTXSID60326692 | |
Description | DSSTox provides a high quality public chemistry resource for supporting improved predictive toxicology. | |
Retrosynthesis Analysis
AI-Powered Synthesis Planning: Our tool employs the Template_relevance Pistachio, Template_relevance Bkms_metabolic, Template_relevance Pistachio_ringbreaker, Template_relevance Reaxys, Template_relevance Reaxys_biocatalysis model, leveraging a vast database of chemical reactions to predict feasible synthetic routes.
One-Step Synthesis Focus: Specifically designed for one-step synthesis, it provides concise and direct routes for your target compounds, streamlining the synthesis process.
Accurate Predictions: Utilizing the extensive PISTACHIO, BKMS_METABOLIC, PISTACHIO_RINGBREAKER, REAXYS, REAXYS_BIOCATALYSIS database, our tool offers high-accuracy predictions, reflecting the latest in chemical research and data.
Strategy Settings
Precursor scoring | Relevance Heuristic |
---|---|
Min. plausibility | 0.01 |
Model | Template_relevance |
Template Set | Pistachio/Bkms_metabolic/Pistachio_ringbreaker/Reaxys/Reaxys_biocatalysis |
Top-N result to add to graph | 6 |
Feasible Synthetic Routes
Q1: What are the primary chemical reactions 3-Bromopentane-2,4-dione is known to undergo?
A1: this compound exhibits interesting reactivity, particularly undergoing deacylation reactions. Research has shown that it can be deacylated in the presence of aromatic compounds using silica-supported Brønsted acids containing sulfonic groups [, ]. This reaction proceeds via the cleavage of the sp3-sp3 carbon-carbon bond, leading to the formation of a carbocation that can then react with the aromatic compound.
Q2: Are there specific applications of this compound in organic synthesis?
A2: While not a common reagent, this compound serves as a useful precursor in synthesizing thienopyridine derivatives []. When reacted with 4,6-diamino-3-cyanopyridine-2(1H)-thione (compound 1 in the study) in a hot potassium hydroxide solution, it forms 2-acetylthienopyridine []. This highlights its potential in constructing heterocyclic compounds with possible biological activity.
Q3: Have the electrochemical properties of this compound derivatives been investigated?
A3: Yes, polarographic studies have been conducted on 3-arylazo-3-bromopentane-2,4-diones []. This research provides insights into the reduction behavior of these compounds, which can be valuable for understanding their reactivity and potential applications in electrochemistry or redox reactions.
Q4: What can be said about the structural characterization of this compound and its derivatives?
A4: Although the provided research snippets don't delve into detailed spectroscopic characterization of this compound itself, they confirm the structure of its derivatives. Techniques like IR, 1H NMR, 13C NMR, and elemental analysis have been used to confirm the structures of various thienopyridine derivatives synthesized using this compound as a starting material []. For deeper insights into the structural properties of this compound, further investigation and spectroscopic analyses would be needed.
Disclaimer and Information on In-Vitro Research Products
Please be aware that all articles and product information presented on BenchChem are intended solely for informational purposes. The products available for purchase on BenchChem are specifically designed for in-vitro studies, which are conducted outside of living organisms. In-vitro studies, derived from the Latin term "in glass," involve experiments performed in controlled laboratory settings using cells or tissues. It is important to note that these products are not categorized as medicines or drugs, and they have not received approval from the FDA for the prevention, treatment, or cure of any medical condition, ailment, or disease. We must emphasize that any form of bodily introduction of these products into humans or animals is strictly prohibited by law. It is essential to adhere to these guidelines to ensure compliance with legal and ethical standards in research and experimentation.