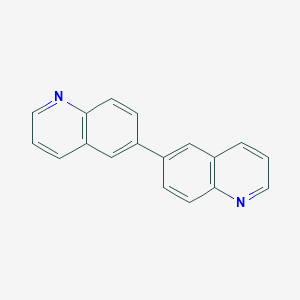
6,6'-Biquinoline
Overview
Description
6,6’-Biquinoline is a heterocyclic aromatic organic compound consisting of two quinoline units connected at the 6th position. This compound is known for its unique structural properties and has been widely studied for its applications in various fields, including chemistry, biology, and materials science.
Scientific Research Applications
6,6’-Biquinoline has a wide range of applications in scientific research:
Medicine: Some derivatives are investigated for their therapeutic potential in treating diseases such as malaria and tuberculosis.
Safety and Hazards
Future Directions
Mechanism of Action
Target of Action
6,6’-Biquinoline, also known as biquinoline, is a heterocyclic compound that has been found to interact with various targets. The primary targets of 6,6’-Biquinoline are copper (II) complexes and the interleukin-6 family of cytokines . Copper (II) complexes are involved in various biological processes, including oxidative stress and DNA damage . The interleukin-6 family of cytokines plays a crucial role in inflammatory responses and immune regulation .
Mode of Action
6,6’-Biquinoline interacts with its targets through various mechanisms. In the case of copper (II) complexes, 6,6’-Biquinoline increases the hydrophobicity of the complexes and provides the ability to act as intercalants in DNA strands through π-stacking type interactions . This interaction leads to increased oxidative stress and DNA damage, which can inhibit tumor cell viability . For the interleukin-6 family of cytokines, 6,6’-Biquinoline-containing compounds have been shown to inhibit the production of these cytokines, thereby modulating inflammatory responses .
Biochemical Pathways
The interaction of 6,6’-Biquinoline with its targets affects various biochemical pathways. The oxidative stress induced by the interaction with copper (II) complexes can lead to apoptosis, a form of programmed cell death . The inhibition of the interleukin-6 family of cytokines can modulate inflammatory responses, which are involved in various diseases, including cancer .
Pharmacokinetics
The physicochemical properties of a molecule, including its lipophilicity and hydrophilicity, can influence its adme properties . These properties can affect the bioavailability of the compound, which is the proportion of the drug that enters the circulation and can have an active effect.
Result of Action
The interaction of 6,6’-Biquinoline with its targets leads to various molecular and cellular effects. The increased oxidative stress and DNA damage caused by the interaction with copper (II) complexes can lead to apoptosis, thereby inhibiting tumor cell viability . The modulation of inflammatory responses through the inhibition of the interleukin-6 family of cytokines can have various effects, depending on the specific cytokine and the context .
Biochemical Analysis
Biochemical Properties
6,6’-Biquinoline plays a crucial role in several biochemical reactions. It interacts with various enzymes, proteins, and other biomolecules, influencing their activity and function. For instance, 6,6’-Biquinoline has been shown to interact with metal ions, forming complexes that can act as enzyme inhibitors or activators. These interactions are essential for understanding the compound’s role in biochemical pathways and its potential therapeutic applications .
Cellular Effects
6,6’-Biquinoline affects various types of cells and cellular processes. It influences cell function by modulating cell signaling pathways, gene expression, and cellular metabolism. Studies have shown that 6,6’-Biquinoline can alter the expression of specific genes involved in cell growth and differentiation. Additionally, it impacts cellular metabolism by interacting with key metabolic enzymes, thereby affecting the overall metabolic flux within the cell .
Molecular Mechanism
The molecular mechanism of 6,6’-Biquinoline involves its binding interactions with biomolecules. It can inhibit or activate enzymes by forming stable complexes with metal ions, which are crucial for the enzyme’s catalytic activity. Furthermore, 6,6’-Biquinoline can influence gene expression by binding to DNA or RNA, thereby modulating the transcription and translation processes. These molecular interactions are fundamental to understanding the compound’s biochemical effects .
Temporal Effects in Laboratory Settings
In laboratory settings, the effects of 6,6’-Biquinoline can change over time. The compound’s stability and degradation are critical factors that influence its long-term effects on cellular function. Studies have shown that 6,6’-Biquinoline is relatively stable under standard laboratory conditions, but its activity can decrease over time due to gradual degradation. Long-term exposure to 6,6’-Biquinoline has been observed to cause changes in cellular function, including alterations in gene expression and metabolic activity .
Dosage Effects in Animal Models
The effects of 6,6’-Biquinoline vary with different dosages in animal models. At low doses, the compound may exhibit beneficial effects, such as enzyme activation or gene expression modulation. At high doses, 6,6’-Biquinoline can cause toxic or adverse effects, including enzyme inhibition and disruption of cellular processes. These dosage-dependent effects are crucial for determining the compound’s therapeutic potential and safety profile .
Metabolic Pathways
6,6’-Biquinoline is involved in several metabolic pathways, interacting with various enzymes and cofactors. It can influence metabolic flux by modulating the activity of key metabolic enzymes, thereby affecting the levels of specific metabolites. Understanding these metabolic pathways is essential for elucidating the compound’s role in cellular metabolism and its potential therapeutic applications .
Transport and Distribution
The transport and distribution of 6,6’-Biquinoline within cells and tissues are mediated by specific transporters and binding proteins. These interactions determine the compound’s localization and accumulation within different cellular compartments. The distribution of 6,6’-Biquinoline can influence its activity and function, as well as its potential therapeutic effects .
Preparation Methods
Synthetic Routes and Reaction Conditions: 6,6’-Biquinoline can be synthesized through several methods. One common approach involves the Skraup reaction, where aniline derivatives react with glycerol and sulfuric acid in the presence of an oxidizing agent. Another method is the Pfitzinger reaction, which involves the reaction of isatin with carbonyl compounds to form quinoline derivatives .
Industrial Production Methods: Industrial production of 6,6’-Biquinoline often involves the use of high-temperature polycondensation reactions. For instance, low-temperature polycondensation of 2,2’-biquinoline-7,7’-diyldimethanamine with terephthaloylbis(3-methoxy-4-hydroxybenzoic) acid dichloride has been used to synthesize polyester amides containing biquinoline units .
Chemical Reactions Analysis
Types of Reactions: 6,6’-Biquinoline undergoes various chemical reactions, including:
Oxidation: It can be oxidized to form quinoline N-oxides.
Reduction: Reduction reactions can convert it into dihydroquinoline derivatives.
Substitution: Electrophilic substitution reactions can introduce various functional groups into the quinoline rings.
Common Reagents and Conditions:
Oxidation: Common oxidizing agents include hydrogen peroxide and peracids.
Reduction: Reducing agents such as sodium borohydride or lithium aluminum hydride are often used.
Substitution: Reagents like halogens, nitrating agents, and sulfonating agents are commonly employed.
Major Products:
Oxidation: Quinoline N-oxides.
Reduction: Dihydroquinoline derivatives.
Substitution: Halogenated, nitrated, or sulfonated quinoline derivatives.
Comparison with Similar Compounds
2,2’-Biquinoline: Another biquinoline isomer with the quinoline units connected at the 2nd position.
4,4’-Biquinoline: This isomer has the quinoline units connected at the 4th position.
8,8’-Biquinoline: In this isomer, the quinoline units are connected at the 8th position.
Uniqueness of 6,6’-Biquinoline: 6,6’-Biquinoline is unique due to its specific connectivity, which imparts distinct electronic and steric properties. This connectivity influences its ability to form stable complexes with transition metals and its interaction with biological targets, making it a valuable compound in various research and industrial applications .
Properties
IUPAC Name |
6-quinolin-6-ylquinoline | |
---|---|---|
Source | PubChem | |
URL | https://pubchem.ncbi.nlm.nih.gov | |
Description | Data deposited in or computed by PubChem | |
InChI |
InChI=1S/C18H12N2/c1-3-15-11-13(5-7-17(15)19-9-1)14-6-8-18-16(12-14)4-2-10-20-18/h1-12H | |
Source | PubChem | |
URL | https://pubchem.ncbi.nlm.nih.gov | |
Description | Data deposited in or computed by PubChem | |
InChI Key |
CCKFFRXAFQLLDF-UHFFFAOYSA-N | |
Source | PubChem | |
URL | https://pubchem.ncbi.nlm.nih.gov | |
Description | Data deposited in or computed by PubChem | |
Canonical SMILES |
C1=CC2=C(C=CC(=C2)C3=CC4=C(C=C3)N=CC=C4)N=C1 | |
Source | PubChem | |
URL | https://pubchem.ncbi.nlm.nih.gov | |
Description | Data deposited in or computed by PubChem | |
Molecular Formula |
C18H12N2 | |
Source | PubChem | |
URL | https://pubchem.ncbi.nlm.nih.gov | |
Description | Data deposited in or computed by PubChem | |
DSSTOX Substance ID |
DTXSID10347372 | |
Record name | 6,6'-Biquinoline | |
Source | EPA DSSTox | |
URL | https://comptox.epa.gov/dashboard/DTXSID10347372 | |
Description | DSSTox provides a high quality public chemistry resource for supporting improved predictive toxicology. | |
Molecular Weight |
256.3 g/mol | |
Source | PubChem | |
URL | https://pubchem.ncbi.nlm.nih.gov | |
Description | Data deposited in or computed by PubChem | |
Solubility |
7.7 [ug/mL] (The mean of the results at pH 7.4) | |
Record name | SID49644700 | |
Source | Burnham Center for Chemical Genomics | |
URL | https://pubchem.ncbi.nlm.nih.gov/bioassay/1996#section=Data-Table | |
Description | Aqueous solubility in buffer at pH 7.4 | |
CAS No. |
612-79-3 | |
Record name | 6,6′-Biquinoline | |
Source | CAS Common Chemistry | |
URL | https://commonchemistry.cas.org/detail?cas_rn=612-79-3 | |
Description | CAS Common Chemistry is an open community resource for accessing chemical information. Nearly 500,000 chemical substances from CAS REGISTRY cover areas of community interest, including common and frequently regulated chemicals, and those relevant to high school and undergraduate chemistry classes. This chemical information, curated by our expert scientists, is provided in alignment with our mission as a division of the American Chemical Society. | |
Explanation | The data from CAS Common Chemistry is provided under a CC-BY-NC 4.0 license, unless otherwise stated. | |
Record name | 6,6'-Biquinoline | |
Source | EPA DSSTox | |
URL | https://comptox.epa.gov/dashboard/DTXSID10347372 | |
Description | DSSTox provides a high quality public chemistry resource for supporting improved predictive toxicology. | |
Retrosynthesis Analysis
AI-Powered Synthesis Planning: Our tool employs the Template_relevance Pistachio, Template_relevance Bkms_metabolic, Template_relevance Pistachio_ringbreaker, Template_relevance Reaxys, Template_relevance Reaxys_biocatalysis model, leveraging a vast database of chemical reactions to predict feasible synthetic routes.
One-Step Synthesis Focus: Specifically designed for one-step synthesis, it provides concise and direct routes for your target compounds, streamlining the synthesis process.
Accurate Predictions: Utilizing the extensive PISTACHIO, BKMS_METABOLIC, PISTACHIO_RINGBREAKER, REAXYS, REAXYS_BIOCATALYSIS database, our tool offers high-accuracy predictions, reflecting the latest in chemical research and data.
Strategy Settings
Precursor scoring | Relevance Heuristic |
---|---|
Min. plausibility | 0.01 |
Model | Template_relevance |
Template Set | Pistachio/Bkms_metabolic/Pistachio_ringbreaker/Reaxys/Reaxys_biocatalysis |
Top-N result to add to graph | 6 |
Feasible Synthetic Routes
Disclaimer and Information on In-Vitro Research Products
Please be aware that all articles and product information presented on BenchChem are intended solely for informational purposes. The products available for purchase on BenchChem are specifically designed for in-vitro studies, which are conducted outside of living organisms. In-vitro studies, derived from the Latin term "in glass," involve experiments performed in controlled laboratory settings using cells or tissues. It is important to note that these products are not categorized as medicines or drugs, and they have not received approval from the FDA for the prevention, treatment, or cure of any medical condition, ailment, or disease. We must emphasize that any form of bodily introduction of these products into humans or animals is strictly prohibited by law. It is essential to adhere to these guidelines to ensure compliance with legal and ethical standards in research and experimentation.