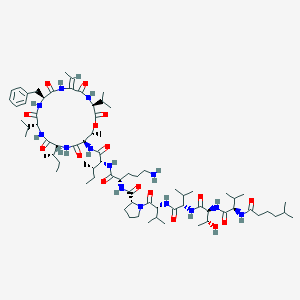
Kahalalide F
Overview
Description
Kahalalide F (KF) is a cyclic depsipeptide derived from the marine mollusk Elysia rufescens and its algal diet Bryopsis spp. . It belongs to the kahalalide family, which includes over 20 structurally related compounds (A–Y) with molecular weights ranging from C31 tetrapeptides to C75 tridecapeptides . KF is distinguished by its unique mechanism of action, targeting lysosomal membranes and inducing oncosis (a necrosis-like cell death) rather than apoptosis . It exhibits potent cytotoxicity against diverse cancer cell lines, including prostate (PC3, IC50 = 0.07 µM), breast (SKBR-3, IC50 = 0.28 µM), and non-small cell lung cancer (A549, IC50 = 0.135 µM) .
Preparation Methods
The synthesis of P-276-00 involves several steps, including the formation of key intermediates and their subsequent reactions. One of the synthetic routes involves the reaction of 1-methyl-4-piperidinone with acetic acid saturated with anhydrous hydrogen chloride gas to yield an intermediate compound . This intermediate undergoes Friedel-Craft acylation with acetic anhydride and boron trifluoride etherate, followed by treatment under basic conditions in dichloromethane to afford the final product . Industrial production methods for P-276-00 are not extensively documented, but they likely involve similar synthetic routes with optimization for large-scale production.
Chemical Reactions Analysis
Hydrolysis of the Lactone Ring
Kahalalide F contains a macrocyclic lactone ring critical for its bioactivity. This ring undergoes pH-dependent hydrolysis to form its linear counterpart, kahalalide G :
The reaction proceeds via nucleophilic attack on the lactone carbonyl, with acidic conditions accelerating degradation. At pH ≤ 1, additional byproducts form due to side-chain modifications, while neutral/basic conditions yield only kahalalide G .
pH-Dependent Degradation Pathways
Degradation studies reveal stability challenges in aqueous environments:
Notably, trifluoroethanol (TFE) promotes supramolecular organization into toroidal nanostructures (~20 nm diameter), as confirmed by cryo-TEM .
Stereochemical Influence on Reactivity
The stereochemistry of Val⁴ and Val⁵ residues dictates both stability and bioactivity:
Synthetic analogs with altered configurations show reduced activity, confirming stereochemistry's role in maintaining the bioactive conformation .
Metabolic Stability
In vitro metabolism studies demonstrate exceptional resistance to enzymatic modification:
The depsipeptide backbone and macrocyclic structure confer resistance to metabolic breakdown, suggesting hepatic clearance plays a minor role .
Synthetic Modifications
Total synthesis efforts have elucidated structure-activity relationships:
Scientific Research Applications
P-276-00 has been extensively studied for its anti-cancer properties. It has shown efficacy in inhibiting the proliferation of various cancer cell lines, including non-small cell lung cancer, head and neck squamous cell carcinoma, and multiple myeloma . In preclinical studies, P-276-00 has demonstrated the ability to induce apoptosis and reduce tumor growth in xenograft models . Additionally, it has been investigated for its potential use in combination therapies with other anti-cancer agents and radiation . Beyond oncology, P-276-00’s role as a CDK inhibitor makes it a valuable tool for studying cell cycle regulation and transcriptional control in various biological contexts .
Mechanism of Action
P-276-00 exerts its effects by inhibiting the activity of cyclin-dependent kinases, particularly CDK1, CDK4, and CDK9 . By binding to the active sites of these kinases, P-276-00 prevents their interaction with cyclins, leading to the inhibition of phosphorylation of key regulatory proteins such as retinoblastoma protein (RB) and E2F transcription factors . This inhibition results in cell cycle arrest at the G1/S phase and induces apoptosis through the activation of the P53 pathway . Additionally, P-276-00 has been shown to reduce the expression of tumor microenvironment proteins such as interleukin-6 and heat shock protein 70 .
Comparison with Similar Compounds
Structural and Functional Comparison with Other Kahalalides
Core Structural Features
All kahalalides share a cyclic depsipeptide backbone with an endocyclic ester bond and N-terminal fatty acid modifications. However, variations in amino acid residues, stereochemistry, and side chains dictate their bioactivity .
Mechanism of Action
- KF : Disrupts lysosomal membranes, inhibits ErbB/HER receptor signaling, and induces oncosis .
- IsoKF (Elisidepsin) : Synthetic analog with enhanced stability; shares KF’s lysosomal targeting but induces oncolysis .
- Kahalalide A: Antimycobacterial activity via unknown mechanisms; linear analogs lose activity, highlighting cyclic structure importance .
Elisidepsin (IsoKF, PM02734)
- Structure : Synthetic KF derivative with modified stereochemistry and alkylation .
- Activity : Broad anti-proliferative effects (IC50 = 0.1–1.0 µM) in gastroesophageal, breast, and NSCLC models .
- Clinical Progress : Phase II for metastatic gastroesophageal cancer; improved pharmacokinetics over KF .
Aza-KF Analogs
- Structure : Replacement of ester bonds with amides .
- Activity : Retain cytotoxicity but require backbone stereochemical precision; side-chain modifications tolerated .
Key Research Findings and Challenges
Selectivity and Toxicity
- KF exhibits 5–40× higher cytotoxicity in cancer vs. normal cells (e.g., HUVECs, IC50 = 1.6–3.1 µM) .
- Dose-limiting hepatotoxicity in clinical trials (transaminase elevation at ≥800 µg/m²) .
Resistance Mechanisms
- KF activity is independent of MDR1 and BCL-2 overexpression, bypassing common resistance pathways .
Biological Activity
Kahalalide F (KF) is a cyclic depsipeptide derived from the marine mollusk Elysia rufescens and the green alga Bryopsis sp. It has garnered attention for its potent biological activities, particularly in the field of oncology. This article explores the biological activity of this compound, focusing on its mechanisms, clinical applications, and research findings.
This compound exhibits a multifaceted mechanism of action primarily targeting cellular membranes and organelles. Key points include:
- Lysosomal Targeting : KF selectively induces cytotoxicity by disrupting lysosomal integrity, leading to cell death through mechanisms independent of caspase activation . This disruption results in mitochondrial membrane potential loss and severe cytoplasmic alterations, including swelling and vacuolization.
- Cell Cycle Arrest : In vitro studies indicate that KF induces cell cycle arrest in the G0-G1 phase across various tumor cell lines, with IC50 values around 1 μmol/L . This effect is particularly noted in prostate, cervical, colon, and non-small cell lung cancer cells.
- Signal Transduction Inhibition : KF inhibits key signaling pathways associated with tumor growth. It has been shown to block the activity of Her2/neu and epidermal growth factor receptors while also affecting the PI3K/Akt signaling pathway. Specifically, KF treatment decreases phosphorylated Akt levels, which are crucial for cell survival and proliferation .
Preclinical Findings
This compound has demonstrated significant antitumor activity in various preclinical models:
- In Vitro : KF showed strong cytotoxic effects against several solid tumor cell lines, including breast (MCF7), colon (CACO-2), and prostate (PC-3) cancer cells. Notably, it was effective against multidrug-resistant cell lines .
- In Vivo : Animal studies revealed that doses of KF administered intraperitoneally resulted in notable treatment-to-control ratios against human tumor xenografts. For instance:
Clinical Trials
This compound has progressed through multiple phases of clinical trials:
- Phase I Trials : A dose-escalation study evaluated safety and pharmacokinetics in patients with advanced solid tumors. The maximum tolerated dose was determined to be 800 μg/m², with a recommended dose for subsequent phases set at 650 μg/m². Some patients experienced stable disease for up to 12.7 months .
- Phase II Trials : Further studies have assessed KF's efficacy in treating advanced melanoma, hepatocellular carcinoma, and non-small cell lung cancer. Results indicated a favorable tolerability profile with no serious adverse events reported . Positive responses were observed in several patients.
Toxicity Profile
While this compound exhibits promising therapeutic effects, it is essential to consider its toxicity profile:
- Dose-Limiting Toxicities : The primary toxicities observed include transient increases in liver transaminases (grade 3/4), which are asymptomatic and manageable with appropriate dosage adjustments. Other noted toxicities include renal tubular effects and central nervous system neurotoxicity .
Summary of Biological Activity
The following table summarizes the biological activity of this compound across various parameters:
Parameter | Details |
---|---|
Source | Marine mollusk Elysia rufescens |
Mechanism of Action | Lysosomal disruption, mitochondrial membrane potential loss |
Cell Cycle Arrest | G0-G1 phase arrest in multiple tumor types |
Key Pathways Affected | Her2/neu inhibition, PI3K/Akt signaling pathway modulation |
Preclinical Efficacy | Effective against various solid tumors; notable activity against resistant lines |
Phase I MTD | 800 μg/m²; recommended dose for Phase II: 650 μg/m² |
Toxicity Profile | Liver toxicity (transaminase elevation), renal and CNS effects |
Q & A
Basic Research Questions
Q. What are the structural characteristics of Kahalalide F, and how are they identified in experimental settings?
this compound is a cyclic depsipeptide with a unique macrocyclic structure. Its identification typically involves nuclear magnetic resonance (NMR) spectroscopy for structural elucidation and liquid chromatography–mass spectrometry (LC-MS) for purity assessment. Researchers must cross-reference spectral data with established literature to confirm identity, particularly focusing on key resonances for amino acid residues like valine and proline derivatives .
Q. What in vitro models are commonly used to study this compound’s cytotoxic mechanisms?
Standard models include human cancer cell lines (e.g., prostate PC-3, breast MDA-MB-231) treated with this compound to assess apoptosis induction via mitochondrial pathways. Methodologies involve flow cytometry for caspase activation, ROS detection assays, and Western blotting for Bcl-2 family protein expression. Dose-response curves are critical to determine IC50 values, requiring triplicate experiments to ensure reproducibility .
Q. How is this compound synthesized in laboratory settings, and what are the key challenges?
Solid-phase peptide synthesis (SPPS) is the primary method, with Fmoc/t-Bu strategies for side-chain protection. Challenges include cyclization efficiency and purification of the macrocyclic product. Researchers must optimize reaction conditions (e.g., coupling reagents, temperature) and employ high-performance liquid chromatography (HPLC) for purification, validated by LC-MS .
Advanced Research Questions
Q. How do researchers address contradictions in this compound’s reported mechanisms of action across studies?
Discrepancies (e.g., lysosomal vs. mitochondrial targeting) require systematic comparison of experimental conditions. For example, differences in cell line lysosomal pH or drug incubation times may explain variability. Meta-analyses of published data, coupled with replication studies using standardized protocols (e.g., fixed exposure durations), are recommended to resolve conflicts .
Q. What methodological considerations are critical when designing in vivo studies for this compound’s antitumor efficacy?
Key factors include:
- Model selection : Xenograft vs. syngeneic models to evaluate immune modulation.
- Dosing regimens : Pharmacokinetic profiling to determine optimal administration routes (e.g., intraperitoneal vs. intravenous).
- Toxicity endpoints : Monitoring liver enzymes (ALT/AST) and renal function markers due to this compound’s hepatotoxic potential. Studies should adhere to ARRIVE guidelines for translational rigor .
Q. How can researchers validate the specificity of this compound’s molecular targets using omics approaches?
Proteomic profiling (e.g., SILAC labeling) and RNA sequencing are used to identify differentially expressed proteins/genes post-treatment. Target validation requires CRISPR/Cas9 knockouts or siRNA silencing of candidate genes (e.g., GRP78 in endoplasmic reticulum stress) followed by rescue experiments to confirm phenotype reversal .
Q. What strategies mitigate batch-to-batch variability in this compound production for preclinical studies?
Implement quality control (QC) protocols:
- Purity thresholds : ≥95% by HPLC.
- Stability testing : Accelerated degradation studies under varying pH/temperature.
- Bioactivity assays : Consistency in IC50 values across batches. Collaborative frameworks for data sharing (e.g., open-access spectral libraries) enhance reproducibility .
Q. Methodological Best Practices
- Data Validation : Use orthogonal assays (e.g., NMR + LC-MS) for compound characterization .
- Ethical Compliance : Obtain IACUC approval for in vivo studies, with explicit justification of sample sizes .
- Literature Synthesis : Leverage systematic reviews to identify knowledge gaps, using tools like PRISMA guidelines .
Properties
IUPAC Name |
(2R)-N-[(2S)-5-amino-1-[[(2R,3S)-1-[[(3S,6Z,9S,12R,15R,18R,19R)-9-benzyl-15-[(2S)-butan-2-yl]-6-ethylidene-19-methyl-2,5,8,11,14,17-hexaoxo-3,12-di(propan-2-yl)-1-oxa-4,7,10,13,16-pentazacyclononadec-18-yl]amino]-3-methyl-1-oxopentan-2-yl]amino]-1-oxopentan-2-yl]-1-[(2R)-2-[[(2S)-2-[[(2S,3R)-3-hydroxy-2-[[(2R)-3-methyl-2-(5-methylhexanoylamino)butanoyl]amino]butanoyl]amino]-3-methylbutanoyl]amino]-3-methylbutanoyl]pyrrolidine-2-carboxamide | |
---|---|---|
Source | PubChem | |
URL | https://pubchem.ncbi.nlm.nih.gov | |
Description | Data deposited in or computed by PubChem | |
InChI |
InChI=1S/C75H124N14O16/c1-20-44(16)59(71(100)88-62-47(19)105-75(104)58(43(14)15)84-63(92)49(22-3)77-65(94)51(37-48-30-24-23-25-31-48)79-67(96)55(40(8)9)81-70(99)60(45(17)21-2)86-73(62)102)85-64(93)50(32-27-35-76)78-66(95)52-33-28-36-89(52)74(103)57(42(12)13)83-69(98)56(41(10)11)82-72(101)61(46(18)90)87-68(97)54(39(6)7)80-53(91)34-26-29-38(4)5/h22-25,30-31,38-47,50-52,54-62,90H,20-21,26-29,32-37,76H2,1-19H3,(H,77,94)(H,78,95)(H,79,96)(H,80,91)(H,81,99)(H,82,101)(H,83,98)(H,84,92)(H,85,93)(H,86,102)(H,87,97)(H,88,100)/b49-22-/t44-,45-,46+,47+,50-,51-,52+,54+,55+,56-,57+,58-,59+,60+,61-,62+/m0/s1 | |
Source | PubChem | |
URL | https://pubchem.ncbi.nlm.nih.gov | |
Description | Data deposited in or computed by PubChem | |
InChI Key |
RCGXNDQKCXNWLO-YUHQQKLOSA-N | |
Source | PubChem | |
URL | https://pubchem.ncbi.nlm.nih.gov | |
Description | Data deposited in or computed by PubChem | |
Canonical SMILES |
CCC(C)C1C(=O)NC(C(=O)NC(C(=O)NC(=CC)C(=O)NC(C(=O)OC(C(C(=O)N1)NC(=O)C(C(C)CC)NC(=O)C(CCCN)NC(=O)C2CCCN2C(=O)C(C(C)C)NC(=O)C(C(C)C)NC(=O)C(C(C)O)NC(=O)C(C(C)C)NC(=O)CCCC(C)C)C)C(C)C)CC3=CC=CC=C3)C(C)C | |
Source | PubChem | |
URL | https://pubchem.ncbi.nlm.nih.gov | |
Description | Data deposited in or computed by PubChem | |
Isomeric SMILES |
CC[C@H](C)[C@@H]1C(=O)N[C@@H](C(=O)N[C@H](C(=O)N/C(=C\C)/C(=O)N[C@H](C(=O)O[C@@H]([C@H](C(=O)N1)NC(=O)[C@@H]([C@@H](C)CC)NC(=O)[C@H](CCCN)NC(=O)[C@H]2CCCN2C(=O)[C@@H](C(C)C)NC(=O)[C@H](C(C)C)NC(=O)[C@H]([C@@H](C)O)NC(=O)[C@@H](C(C)C)NC(=O)CCCC(C)C)C)C(C)C)CC3=CC=CC=C3)C(C)C | |
Source | PubChem | |
URL | https://pubchem.ncbi.nlm.nih.gov | |
Description | Data deposited in or computed by PubChem | |
Molecular Formula |
C75H124N14O16 | |
Source | PubChem | |
URL | https://pubchem.ncbi.nlm.nih.gov | |
Description | Data deposited in or computed by PubChem | |
DSSTOX Substance ID |
DTXSID301031233 | |
Record name | Kahalalide F | |
Source | EPA DSSTox | |
URL | https://comptox.epa.gov/dashboard/DTXSID301031233 | |
Description | DSSTox provides a high quality public chemistry resource for supporting improved predictive toxicology. | |
Molecular Weight |
1477.9 g/mol | |
Source | PubChem | |
URL | https://pubchem.ncbi.nlm.nih.gov | |
Description | Data deposited in or computed by PubChem | |
CAS No. |
149204-42-2 | |
Record name | Kahalalide F | |
Source | ChemIDplus | |
URL | https://pubchem.ncbi.nlm.nih.gov/substance/?source=chemidplus&sourceid=0149204422 | |
Description | ChemIDplus is a free, web search system that provides access to the structure and nomenclature authority files used for the identification of chemical substances cited in National Library of Medicine (NLM) databases, including the TOXNET system. | |
Record name | Kahalalide F | |
Source | EPA DSSTox | |
URL | https://comptox.epa.gov/dashboard/DTXSID301031233 | |
Description | DSSTox provides a high quality public chemistry resource for supporting improved predictive toxicology. | |
Record name | KAHALALIDE F | |
Source | FDA Global Substance Registration System (GSRS) | |
URL | https://gsrs.ncats.nih.gov/ginas/app/beta/substances/BQD6E2ZXEA | |
Description | The FDA Global Substance Registration System (GSRS) enables the efficient and accurate exchange of information on what substances are in regulated products. Instead of relying on names, which vary across regulatory domains, countries, and regions, the GSRS knowledge base makes it possible for substances to be defined by standardized, scientific descriptions. | |
Explanation | Unless otherwise noted, the contents of the FDA website (www.fda.gov), both text and graphics, are not copyrighted. They are in the public domain and may be republished, reprinted and otherwise used freely by anyone without the need to obtain permission from FDA. Credit to the U.S. Food and Drug Administration as the source is appreciated but not required. | |
Retrosynthesis Analysis
AI-Powered Synthesis Planning: Our tool employs the Template_relevance Pistachio, Template_relevance Bkms_metabolic, Template_relevance Pistachio_ringbreaker, Template_relevance Reaxys, Template_relevance Reaxys_biocatalysis model, leveraging a vast database of chemical reactions to predict feasible synthetic routes.
One-Step Synthesis Focus: Specifically designed for one-step synthesis, it provides concise and direct routes for your target compounds, streamlining the synthesis process.
Accurate Predictions: Utilizing the extensive PISTACHIO, BKMS_METABOLIC, PISTACHIO_RINGBREAKER, REAXYS, REAXYS_BIOCATALYSIS database, our tool offers high-accuracy predictions, reflecting the latest in chemical research and data.
Strategy Settings
Precursor scoring | Relevance Heuristic |
---|---|
Min. plausibility | 0.01 |
Model | Template_relevance |
Template Set | Pistachio/Bkms_metabolic/Pistachio_ringbreaker/Reaxys/Reaxys_biocatalysis |
Top-N result to add to graph | 6 |
Feasible Synthetic Routes
Disclaimer and Information on In-Vitro Research Products
Please be aware that all articles and product information presented on BenchChem are intended solely for informational purposes. The products available for purchase on BenchChem are specifically designed for in-vitro studies, which are conducted outside of living organisms. In-vitro studies, derived from the Latin term "in glass," involve experiments performed in controlled laboratory settings using cells or tissues. It is important to note that these products are not categorized as medicines or drugs, and they have not received approval from the FDA for the prevention, treatment, or cure of any medical condition, ailment, or disease. We must emphasize that any form of bodily introduction of these products into humans or animals is strictly prohibited by law. It is essential to adhere to these guidelines to ensure compliance with legal and ethical standards in research and experimentation.