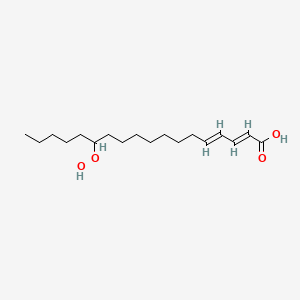
13-Hydroperoxyoctadecadienoic acid
- Click on QUICK INQUIRY to receive a quote from our team of experts.
- With the quality product at a COMPETITIVE price, you can focus more on your research.
Overview
Description
13-Hydroperoxyoctadecadienoic acid (13-HPODE) is a lipid hydroperoxide derived from the oxidation of linoleic acid (LA) via 15-lipoxygenase (15-LOX) . It plays dual roles in cellular processes, acting as both a pro-oxidant and a modulator of redox-sensitive pathways. Structurally, it contains a hydroperoxy group at the 13th carbon and conjugated dienes at positions 9 and 11 (9Z,11E configuration) . Key biological effects include:
- PPAR Activation: 13-HPODE enhances peroxisome proliferator-activated receptor (PPAR) signaling, particularly PPARα and PPARγ, influencing lipid metabolism and inflammation .
- Antioxidant Defense: Upregulates detoxification pathways (e.g., cytochrome P450, glutathione metabolism) to counteract oxidative stress .
- Gene Regulation: Modulates genes involved in proliferation (e.g., downregulates CCN1/2, PIM1), differentiation (e.g., upregulates CEBPA/PB), and inflammation (e.g., downregulates CXCL1/3) .
Preparation Methods
Synthetic Routes and Reaction Conditions: 13-Hydroperoxyoctadecadienoic acid can be synthesized through the lipoxygenase-catalyzed oxidation of linoleic acid. This process involves the use of lipoxygenase enzymes, which catalyze the hydroperoxidation of polyunsaturated fatty acids. The reaction conditions typically include a solvent-free system and the addition of surfactants to improve the reaction yield .
Industrial Production Methods: In an industrial setting, the production of this compound involves an enzyme cascade consisting of lipoxygenase, lipase, and catalase. This one-pot synthesis method starts with safflower oil and optimizes hydroperoxidation using lipoxygenase 1 from Glycine max (LOX-1). The addition of green surfactants and continuous oxygen generation enhances the efficiency of the process .
Chemical Reactions Analysis
Types of Reactions: 13-Hydroperoxyoctadecadienoic acid undergoes various chemical reactions, including oxidation, reduction, and substitution. It is particularly prone to oxidative modification, which can lead to the formation of protein carbonyl derivatives and the loss of protein sulfhydryl groups .
Common Reagents and Conditions: Common reagents used in the reactions of this compound include antioxidants, reducing agents, and various enzymes. The reaction conditions often involve specific pH levels, temperatures, and the presence of surfactants to facilitate the reactions .
Major Products: The major products formed from the reactions of this compound include aldehydes, ketones, and other oxygenated compounds. These products result from the complex free radical reactions involved in lipid peroxidation .
Scientific Research Applications
Gene Expression Modulation
Research has demonstrated that 13-HPODE significantly alters gene expression in intestinal epithelial cells (Caco-2). A study using RNA sequencing revealed that treatment with 13-HPODE resulted in the identification of 3,094 differentially expressed genes (DEGs), suggesting its role in modulating lipid metabolism, detoxification pathways, and cellular stress responses . Notably, it enhances pathways related to steroid hormone biosynthesis and peroxisome proliferator-activated receptor signaling, which are crucial for lipid homeostasis and inflammation regulation.
Impact on Mitochondrial Function
13-HPODE has been implicated in mitochondrial dysfunction. Its treatment led to downregulation of oxidative phosphorylation pathways, indicating potential oxidative damage to mitochondrial function . This effect may contribute to various metabolic disorders and diseases linked to oxidative stress.
Cancer Research
The compound's ability to influence cellular proliferation and apoptosis is noteworthy in cancer research. 13-HPODE has been shown to promote a tumorigenic environment by enhancing amino acid metabolism while downregulating genes associated with cell cycle progression . This dual action suggests that 13-HPODE could be a target for therapeutic strategies aimed at modulating cancer cell behavior.
Inflammatory Diseases
In studies focusing on chronic inflammation, 13-HPODE has been linked to the activation of nuclear factor kappa B (NF-κB), a key regulator of inflammatory responses . This suggests that 13-HPODE may play a role in the pathology of inflammatory diseases such as rheumatoid arthritis and inflammatory bowel disease.
Protein Modification
In food science, 13-HPODE has been studied for its effects on protein structure and functionality. For instance, when rice protein was incubated with 13-HPODE, it resulted in protein carbonylation and alterations in secondary structure, negatively impacting solubility and functional properties such as foaming capacity . This modification could have implications for food texture and stability.
Lipid Peroxidation Studies
As a primary product of lipid peroxidation, 13-HPODE serves as a model compound for studying oxidative degradation processes in food products. Its presence can indicate the extent of lipid oxidation, which is crucial for assessing food quality and shelf life.
Case Studies
Mechanism of Action
The mechanism of action of 13-Hydroperoxyoctadecadienoic acid involves its interaction with various molecular targets and pathways. It activates the nuclear factor-kB (NF-kB) signaling pathway, leading to the transcriptional regulation of inflammatory genes in vascular smooth muscle cells. This activation is mediated by the Ras and mitogen-activated protein kinase (MAPK) pathways . Additionally, this compound induces oxidative stress and the formation of reactive oxygen species, contributing to its biological effects .
Comparison with Similar Compounds
Comparison with Structurally and Functionally Related Compounds
13-HPODE vs. 13-Hydroxyoctadecadienoic Acid (13-HODE)
13-HPODE vs. 9-Hydroperoxyoctadecadienoic Acid (9-HPODE)
13-HPODE vs. 15-Hydroperoxyeicosatetraenoic Acid (15-HPETE)
13-HPODE vs. Hydrogen Peroxide (H₂O₂)
Key Research Findings and Controversies
- Dual Role in Cancer : 13-HPODE suppresses proliferation via MYC downregulation but may promote tumorigenesis by enhancing SRC oncogene and lipid droplet formation (PLIN2) .
- PPAR Signaling : While PPAR activation improves metabolic homeostasis, chronic activation may drive cancer progression .
- Inflammation Modulation: Contrasting reports note 13-HPODE’s anti-inflammatory effects (e.g., CXCL1/3 downregulation ) versus pro-inflammatory outcomes (e.g., ICAM-1 induction ), likely context-dependent.
Biological Activity
13-Hydroperoxyoctadecadienoic acid (13-HPODE) is a lipid peroxidation product derived from linoleic acid, a polyunsaturated fatty acid prevalent in the human diet. This compound has garnered attention due to its significant biological activities and implications in various health conditions, including inflammation, cancer, and metabolic disorders. This article explores the biological activity of 13-HPODE, focusing on its effects on cellular processes, gene expression, and potential therapeutic applications.
Gene Expression Modulation
Research indicates that 13-HPODE alters gene expression in intestinal epithelial cells, notably Caco-2 cells. A study employing RNA sequencing revealed that treatment with 100 µM of 13-HPODE resulted in the identification of 3,094 differentially expressed genes (DEGs) compared to untreated controls. Key pathways affected include:
- Lipid Metabolism : Enhanced pathways related to steroid hormone biosynthesis and peroxisome proliferator-activated receptor (PPAR) signaling were observed. This suggests that 13-HPODE may influence lipid uptake and transport mechanisms significantly .
- Detoxification Pathways : Upregulation of cytochrome P450 enzymes and glutathione metabolism indicates a potential role in detoxifying reactive oxygen species (ROS) and other harmful metabolites .
- Cell Cycle Regulation : Downregulation of genes associated with the cell cycle and DNA repair mechanisms suggests that 13-HPODE may inhibit cellular proliferation, potentially contributing to tumorigenic environments .
Impact on Cellular Functions
The effects of 13-HPODE extend beyond gene expression to impact various cellular functions:
- Mitochondrial Dysfunction : Treatment with 13-HPODE has been linked to disrupted mitochondrial function, characterized by downregulation of oxidative phosphorylation pathways. This disruption may lead to increased oxidative stress and contribute to disease progression .
- Protein Carbonylation : In vitro studies have shown that incubation of proteins with 13-HPODE leads to carbonylation and loss of functional groups, which can impair protein function and stability .
- Inflammatory Response : While some inflammatory genes are downregulated following treatment with 13-HPODE, the overall impact on inflammatory pathways remains complex and context-dependent .
Study 1: Effects on Caco-2 Cells
A notable study investigated the effects of 13-HPODE on Caco-2 cells, which serve as a model for intestinal epithelium. The results demonstrated significant alterations in gene expression related to lipid metabolism and detoxification pathways. Notably, the study highlighted the compound's potential role in promoting absorptive cell differentiation while reducing proliferation through suppression of cell cycle-related pathways .
Study 2: Protein Modification in Rice
Another study focused on the impact of 13-HPODE on rice protein during aging. It was found that exposure to increasing concentrations of 13-HPODE resulted in structural modifications of proteins, leading to decreased solubility and functional properties such as foaming capacity. This suggests that lipid peroxidation products can adversely affect protein functionality in food systems .
Data Summary
Q & A
Q. What are the primary enzymatic and non-enzymatic pathways for synthesizing 13-HPODE in biological systems?
Basic
13-HPODE is synthesized via two main pathways:
- Enzymatic oxidation : Lipoxygenases (LOXs), particularly 13-LOX isoforms, catalyze the stereospecific oxidation of linoleic acid at carbon 13, producing 13(S)-HPODE .
- Non-enzymatic autoxidation : Under oxidative stress, free radicals mediate the peroxidation of linoleic acid, generating a racemic mixture of 13-HPODE .
Methodological Note : To distinguish enzymatic vs. non-enzymatic products, use chiral-phase HPLC to confirm stereochemistry (S-configuration in enzymatic synthesis vs. racemic mixtures in autoxidation) .
Q. How can researchers detect and quantify 13-HPODE in complex biological matrices?
Basic
Key analytical strategies include:
- UV spectroscopy : Detect conjugated dienes at 234 nm, characteristic of 13-HPODE .
- Chromatography : Reverse-phase HPLC or GC-MS (after derivatization) for separation and quantification .
Advanced : For low-abundance samples, employ isotope dilution mass spectrometry (IDMS) with deuterated internal standards to improve accuracy and account for matrix effects .
Q. What experimental models are suitable for studying 13-HPODE-induced protein carbonylation and functional alterations?
Advanced
- In vitro systems : Incubate purified proteins (e.g., rice protein or albumin) with 13-HPODE under controlled oxygen levels. Monitor structural changes via circular dichroism (CD) for secondary structure shifts (e.g., α-helix to β-sheet transitions) .
- Biomarker detection : Use immunoblotting or LC-MS to identify Nε-(Hexanonyl)lysine (HEL), a stable adduct formed by 13-HPODE-protein interactions .
Q. How does 13-HPODE contribute to oxidative stress and cytotoxicity in endothelial cells?
Advanced
- Mechanism : 13-HPODE decomposes into reactive aldehydes (e.g., 4-hydroxynonenal) via transition metal-catalyzed reactions, leading to mitochondrial dysfunction and ROS overproduction .
- Experimental design : Treat endothelial cells with 13-HPODE (10–50 µM) and measure ROS using DCFH-DA fluorescence. Validate with antioxidants like Mito-Q to confirm mitochondrial targeting .
Q. What are the conflicting roles of 13-HPODE in inflammation and signaling pathways?
Advanced
- Pro-inflammatory effects : 13-HPODE activates NF-κB and upregulates COX-2, enhancing prostaglandin synthesis in macrophages .
- Paradoxical signaling : At low concentrations, 13-HPODE may regulate redox-sensitive transcription factors (e.g., Nrf2), suggesting context-dependent roles .
Data contradiction : Compare 13-HPODE with its reduced metabolite, 13-HODE, which exhibits weaker pro-inflammatory activity despite structural similarity .
Q. How can researchers address challenges in stabilizing 13-HPODE during experimental workflows?
Methodological
- Storage : Store 13-HPODE in inert solvents (e.g., ethanol) at -80°C under argon to prevent decomposition .
- Handling : Avoid transition metals (Fe²⁺, Cu²⁺) in buffers; use chelators like EDTA to inhibit metal-catalyzed degradation .
Q. What strategies exist for targeting 13-HPODE in therapeutic interventions for atherosclerosis?
Advanced
- Inhibition of formation : Develop LOX inhibitors (e.g., NDGA) to block 13-HPODE synthesis in oxidized LDL .
- Neutralization : Use scavengers like glutathione peroxidase to reduce 13-HPODE to 13-HODE, mitigating its pro-atherogenic effects .
Q. How does 13-HPODE compare to other lipid peroxidation products (e.g., MDA, HNE) in cellular damage assays?
Advanced
- Primary vs. secondary products : 13-HPODE (primary) directly modifies proteins, while MDA and HNE (secondary) form cross-links with DNA and proteins .
- Experimental comparison : Co-treat cells with 13-HPODE and HNE; quantify adducts via LC-MS to assess relative contributions to cytotoxicity .
Q. What in vivo models are appropriate for studying dietary exposure to 13-HPODE?
Advanced
- Rodent models : Feed mice thermally oxidized oils rich in 13-HPODE and monitor hepatic lipid peroxidation markers (e.g., HEL adducts) .
- Limitations : Consider species-specific LOX activity; rabbits and ApoE⁻/⁻ mice better replicate human atherosclerosis .
Q. How can researchers resolve discrepancies in 13-HPODE's reported bioactivity across studies?
Methodological
Properties
CAS No. |
28040-10-0 |
---|---|
Molecular Formula |
C18H32O4 |
Molecular Weight |
312.4 g/mol |
IUPAC Name |
(2E,4E)-13-hydroperoxyoctadeca-2,4-dienoic acid |
InChI |
InChI=1S/C18H32O4/c1-2-3-11-14-17(22-21)15-12-9-7-5-4-6-8-10-13-16-18(19)20/h8,10,13,16-17,21H,2-7,9,11-12,14-15H2,1H3,(H,19,20)/b10-8+,16-13+ |
InChI Key |
QEUHXYPYKZKYFW-ZQHUEGGHSA-N |
Isomeric SMILES |
CCCCCC(CCCCCCC/C=C/C=C/C(=O)O)OO |
Canonical SMILES |
CCCCCC(CCCCCCCC=CC=CC(=O)O)OO |
Origin of Product |
United States |
Disclaimer and Information on In-Vitro Research Products
Please be aware that all articles and product information presented on BenchChem are intended solely for informational purposes. The products available for purchase on BenchChem are specifically designed for in-vitro studies, which are conducted outside of living organisms. In-vitro studies, derived from the Latin term "in glass," involve experiments performed in controlled laboratory settings using cells or tissues. It is important to note that these products are not categorized as medicines or drugs, and they have not received approval from the FDA for the prevention, treatment, or cure of any medical condition, ailment, or disease. We must emphasize that any form of bodily introduction of these products into humans or animals is strictly prohibited by law. It is essential to adhere to these guidelines to ensure compliance with legal and ethical standards in research and experimentation.