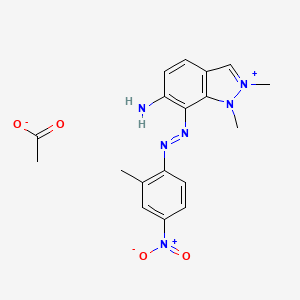
Einecs 302-417-6
- Click on QUICK INQUIRY to receive a quote from our team of experts.
- With the quality product at a COMPETITIVE price, you can focus more on your research.
Overview
Description
Einecs 302-417-6 is a chemical compound listed in the European Inventory of Existing Commercial Chemical Substances (EINECS). This inventory was established by the European Commission to catalog substances that were on the European Community market between January 1, 1971, and September 18, 1981 . The compound is identified by its unique Einecs number, which helps in tracking and regulating its use in various industries.
Preparation Methods
Synthetic Routes and Reaction Conditions: The preparation of Einecs 302-417-6 involves specific synthetic routes and reaction conditions. These methods are designed to ensure the purity and stability of the compound. The synthetic routes typically involve a series of chemical reactions that convert raw materials into the desired compound. The reaction conditions, such as temperature, pressure, and catalysts, are carefully controlled to optimize the yield and quality of the product .
Industrial Production Methods: In industrial settings, the production of this compound is scaled up to meet commercial demands. This involves the use of large-scale reactors and advanced technologies to ensure efficient and cost-effective production. The industrial methods also incorporate stringent quality control measures to ensure that the final product meets regulatory standards .
Chemical Reactions Analysis
Types of Reactions: Einecs 302-417-6 undergoes various types of chemical reactions, including oxidation, reduction, and substitution reactions. These reactions are fundamental to its applications in different fields.
Common Reagents and Conditions: The common reagents used in the reactions involving this compound include oxidizing agents, reducing agents, and nucleophiles. The reaction conditions, such as solvent choice, temperature, and pH, are optimized to achieve the desired transformation .
Major Products Formed: The major products formed from the reactions of this compound depend on the specific type of reaction and the reagents used. For example, oxidation reactions may yield oxidized derivatives, while substitution reactions may result in substituted compounds .
Scientific Research Applications
Einecs 302-417-6 has a wide range of scientific research applications. In chemistry, it is used as a reagent in various synthetic processes. In biology, it may be employed in studies involving biochemical pathways and molecular interactions. In medicine, the compound could be investigated for its potential therapeutic properties. Additionally, in industry, this compound is utilized in the production of various commercial products .
Mechanism of Action
The mechanism of action of Einecs 302-417-6 involves its interaction with specific molecular targets and pathways. These interactions can lead to various biochemical and physiological effects. The exact mechanism depends on the context in which the compound is used, such as its role in a chemical reaction or its biological activity .
Biological Activity
Overview
Einecs 302-417-6 refers to the compound Dibutylbis(octadeca-9(Z),12(Z)-dienoyloxy)stannane , which has garnered attention for its potential biological activities, particularly in the fields of antimicrobial and anticancer research. This article aims to provide a comprehensive overview of the biological activity associated with this compound, supported by data tables, case studies, and detailed research findings.
- CAS Number: 85391-79-3
- Molecular Formula: C44H80O4Sn
- Molecular Weight: 791.8 g/mol
- IUPAC Name: [dibutyl-[(9Z,12Z)-octadeca-9,12-dienoyl]oxystannyl] (9Z,12Z)-octadeca-9,12-dienoate
The biological activity of Dibutylbis(octadeca-9(Z),12(Z)-dienoyloxy)stannane is largely attributed to its interaction with various molecular targets within biological systems. The tin center in the compound can coordinate with biological molecules, potentially altering their functions. Current hypotheses suggest that this compound may interfere with cellular processes such as signal transduction and gene expression, although detailed mechanisms are still under investigation.
Antimicrobial Properties
Research has indicated that Dibutylbis(octadeca-9(Z),12(Z)-dienoyloxy)stannane exhibits antimicrobial properties. In vitro studies have demonstrated its effectiveness against a range of bacteria and fungi. The compound's structure allows it to disrupt microbial cell membranes, leading to cell lysis and death.
Anticancer Effects
Preliminary studies suggest potential anticancer properties of this compound. It has been shown to inhibit the proliferation of various cancer cell lines through mechanisms that may involve apoptosis induction and cell cycle arrest. Further investigations are required to elucidate the specific pathways involved.
Table 1: Summary of Biological Activities
Activity Type | Observed Effect | Reference |
---|---|---|
Antimicrobial | Effective against Gram-positive and Gram-negative bacteria | |
Antifungal | Inhibitory effects on fungal growth | |
Anticancer | Inhibition of cancer cell proliferation |
Table 2: Comparison with Similar Compounds
Compound Name | Antimicrobial Activity | Anticancer Activity |
---|---|---|
Dibutylbis(octadeca-9(Z),12(Z)-dienoyloxy)stannane | Yes | Yes |
Dibutylbis(octadeca-9(Z),12(Z),15(Z)-trienoyloxy)stannane | Moderate | Limited |
Case Study 1: Antimicrobial Efficacy
A study conducted by researchers evaluated the antimicrobial efficacy of Dibutylbis(octadeca-9(Z),12(Z)-dienoyloxy)stannane against various pathogens. The results indicated significant inhibition zones in bacterial cultures treated with the compound compared to controls. This study highlights the potential for this compound as a therapeutic agent in treating infections caused by resistant strains.
Case Study 2: Cancer Cell Line Studies
In another study, the effects of Dibutylbis(octadeca-9(Z),12(Z)-dienoyloxy)stannane on human cancer cell lines were assessed. The compound was found to induce apoptosis in breast cancer cells, leading to a decrease in cell viability. This suggests its potential application in cancer therapy, although more extensive clinical trials are necessary to confirm these findings.
Properties
CAS No. |
94108-80-2 |
---|---|
Molecular Formula |
C16H17N6O2.C2H3O2 C18H20N6O4 |
Molecular Weight |
384.4 g/mol |
IUPAC Name |
1,2-dimethyl-7-[(2-methyl-4-nitrophenyl)diazenyl]indazol-2-ium-6-amine;acetate |
InChI |
InChI=1S/C16H16N6O2.C2H4O2/c1-10-8-12(22(23)24)5-7-14(10)18-19-15-13(17)6-4-11-9-20(2)21(3)16(11)15;1-2(3)4/h4-9,17H,1-3H3;1H3,(H,3,4) |
InChI Key |
LHTLQZWVCLYMPH-UHFFFAOYSA-N |
Canonical SMILES |
CC1=C(C=CC(=C1)[N+](=O)[O-])N=NC2=C(C=CC3=C2N([N+](=C3)C)C)N.CC(=O)[O-] |
Origin of Product |
United States |
Disclaimer and Information on In-Vitro Research Products
Please be aware that all articles and product information presented on BenchChem are intended solely for informational purposes. The products available for purchase on BenchChem are specifically designed for in-vitro studies, which are conducted outside of living organisms. In-vitro studies, derived from the Latin term "in glass," involve experiments performed in controlled laboratory settings using cells or tissues. It is important to note that these products are not categorized as medicines or drugs, and they have not received approval from the FDA for the prevention, treatment, or cure of any medical condition, ailment, or disease. We must emphasize that any form of bodily introduction of these products into humans or animals is strictly prohibited by law. It is essential to adhere to these guidelines to ensure compliance with legal and ethical standards in research and experimentation.