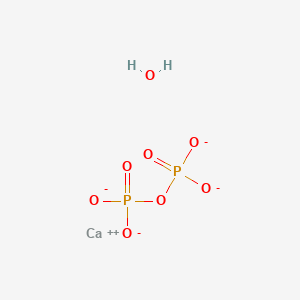
Calcium diphosphate dihydrate
- Click on QUICK INQUIRY to receive a quote from our team of experts.
- With the quality product at a COMPETITIVE price, you can focus more on your research.
Overview
Description
Calcium diphosphate dihydrate, also known as dicalcium phosphate dihydrate, is a calcium phosphate compound with the chemical formula CaHPO₄·2H₂O. It is commonly referred to as brushite in its mineral form. This compound is widely used in various industries, including food, pharmaceuticals, and agriculture, due to its beneficial properties such as being a source of calcium and phosphate.
Preparation Methods
Synthetic Routes and Reaction Conditions
Calcium diphosphate dihydrate can be synthesized through the neutralization of calcium hydroxide with phosphoric acid. The reaction is typically carried out at a temperature of around 60°C, resulting in the precipitation of the dihydrate form: [ \text{H}_3\text{PO}_4 + \text{Ca(OH)}_2 \rightarrow \text{CaHPO}_4 \cdot 2\text{H}_2\text{O} ]
Another method involves treating calcium chloride with ammonium phosphate: [ \text{CaCl}_2 + (\text{NH}_4)_2\text{HPO}_4 \rightarrow \text{CaHPO}_4 \cdot 2\text{H}_2\text{O} + 2\text{NH}_4\text{Cl} ]
Industrial Production Methods
In industrial settings, the production of this compound often involves a continuous process where calcium chloride is treated with ammonium phosphate to form the dihydrate. The resulting slurry is then heated to around 65-70°C to form anhydrous calcium diphosphate as a crystalline precipitate .
Chemical Reactions Analysis
Types of Reactions
Calcium diphosphate dihydrate undergoes various chemical reactions, including:
Neutralization: Reacts with acids to form soluble calcium salts and phosphoric acid.
Hydrolysis: In aqueous solutions, it can hydrolyze to form calcium ions and phosphate ions.
Common Reagents and Conditions
Acids: Hydrochloric acid, sulfuric acid, and nitric acid are commonly used to react with this compound.
Conditions: Reactions are typically carried out at room temperature or slightly elevated temperatures.
Major Products
Calcium Salts: Such as calcium chloride when reacted with hydrochloric acid.
Phosphoric Acid: Formed during the neutralization reactions.
Scientific Research Applications
Calcium diphosphate dihydrate has numerous applications in scientific research:
Chemistry: Used as a reagent in various chemical reactions and as a precursor for other calcium phosphate compounds.
Biology: Studied for its role in biomineralization processes and as a model compound for understanding calcium phosphate formation in biological systems.
Industry: Employed in the production of fertilizers and as a feed additive in livestock nutrition.
Mechanism of Action
The mechanism of action of calcium diphosphate dihydrate involves its dissociation into calcium and phosphate ions in aqueous environments. These ions play crucial roles in various physiological processes:
Calcium Ions: Essential for bone mineralization and maintaining bone density.
Phosphate Ions: Involved in energy metabolism and cellular signaling pathways.
In the stomach, this compound reacts with hydrochloric acid to neutralize the pH, providing a source of calcium and phosphate ions for remineralization of teeth and bone homeostasis .
Comparison with Similar Compounds
Calcium diphosphate dihydrate can be compared with other calcium phosphate compounds:
Monocalcium Phosphate Monohydrate (Ca(H₂PO₄)₂·H₂O): Used as a leavening agent in baking.
Tricalcium Phosphate (Ca₃(PO₄)₂): Used as an anti-caking agent and in bone graft materials.
Hydroxyapatite (Ca₁₀(PO₄)₆(OH)₂): The primary mineral component of bone and teeth, used in medical implants.
Each of these compounds has unique properties and applications, with this compound being particularly valued for its solubility and bioavailability.
Properties
CAS No. |
17031-92-4 |
---|---|
Molecular Formula |
CaH2O8P2-2 |
Molecular Weight |
232.04 g/mol |
IUPAC Name |
calcium;phosphonato phosphate;hydrate |
InChI |
InChI=1S/Ca.H4O7P2.H2O/c;1-8(2,3)7-9(4,5)6;/h;(H2,1,2,3)(H2,4,5,6);1H2/q+2;;/p-4 |
InChI Key |
LOGGAGJVXOASPM-UHFFFAOYSA-J |
Canonical SMILES |
O.[O-]P(=O)([O-])OP(=O)([O-])[O-].[Ca+2] |
Origin of Product |
United States |
Disclaimer and Information on In-Vitro Research Products
Please be aware that all articles and product information presented on BenchChem are intended solely for informational purposes. The products available for purchase on BenchChem are specifically designed for in-vitro studies, which are conducted outside of living organisms. In-vitro studies, derived from the Latin term "in glass," involve experiments performed in controlled laboratory settings using cells or tissues. It is important to note that these products are not categorized as medicines or drugs, and they have not received approval from the FDA for the prevention, treatment, or cure of any medical condition, ailment, or disease. We must emphasize that any form of bodily introduction of these products into humans or animals is strictly prohibited by law. It is essential to adhere to these guidelines to ensure compliance with legal and ethical standards in research and experimentation.