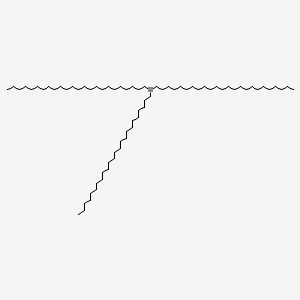
Aluminum, tritetracosyl-
- Click on QUICK INQUIRY to receive a quote from our team of experts.
- With the quality product at a COMPETITIVE price, you can focus more on your research.
Overview
Description
Aluminum, tritetracosyl- is an organoaluminum compound characterized by the presence of long-chain alkyl groups attached to the aluminum atom. This compound is part of a broader class of organoaluminum compounds, which are known for their diverse applications in organic synthesis and industrial processes.
Preparation Methods
Synthetic Routes and Reaction Conditions: The preparation of Aluminum, tritetracosyl- typically involves the reaction of aluminum with tetracosyl halides under controlled conditions. The reaction is carried out in an inert atmosphere to prevent oxidation and hydrolysis. The general reaction can be represented as: [ \text{Al} + 3 \text{R-Cl} \rightarrow \text{Al®}_3 + 3 \text{HCl} ] where R represents the tetracosyl group.
Industrial Production Methods: Industrial production of Aluminum, tritetracosyl- follows similar synthetic routes but on a larger scale. The process involves the use of high-purity aluminum and tetracosyl halides, with the reaction being conducted in large reactors equipped with inert gas purging systems to maintain an oxygen-free environment.
Types of Reactions:
Oxidation: Aluminum, tritetracosyl- can undergo oxidation reactions, forming aluminum oxides and other oxidation products.
Reduction: This compound can be reduced under specific conditions, often involving the use of strong reducing agents.
Substitution: It can participate in substitution reactions where the tetracosyl groups are replaced by other functional groups.
Common Reagents and Conditions:
Oxidation: Oxygen or other oxidizing agents under controlled temperature and pressure.
Reduction: Lithium aluminum hydride (LiAlH4) or other strong reducing agents.
Substitution: Halogenating agents or other electrophiles.
Major Products:
Oxidation: Aluminum oxides and hydroxides.
Reduction: Reduced aluminum species.
Substitution: Various substituted aluminum compounds.
Scientific Research Applications
Aluminum, tritetracosyl- has a wide range of applications in scientific research:
Chemistry: Used as a catalyst in organic synthesis, particularly in polymerization reactions.
Biology: Investigated for its potential use in drug delivery systems due to its ability to form stable complexes with various biomolecules.
Medicine: Explored for its potential in targeted drug delivery and as an adjuvant in vaccines.
Industry: Utilized in the production of high-performance materials and as a component in specialty coatings and lubricants.
Mechanism of Action
The mechanism of action of Aluminum, tritetracosyl- involves its ability to form stable complexes with various substrates. The aluminum atom acts as a Lewis acid, accepting electron pairs from donor molecules. This interaction facilitates various chemical transformations, making it a valuable catalyst in organic synthesis. The long tetracosyl chains provide steric hindrance, influencing the reactivity and selectivity of the compound.
Comparison with Similar Compounds
Triethylaluminum: Another organoaluminum compound with shorter ethyl groups.
Trimethylaluminum: Similar structure but with methyl groups instead of tetracosyl groups.
Triisobutylaluminum: Contains isobutyl groups.
Comparison:
Uniqueness: The long tetracosyl chains in Aluminum, tritetracosyl- provide unique steric and electronic properties, making it distinct from other organoaluminum compounds. This uniqueness allows for specific applications in catalysis and material science that are not achievable with shorter-chain analogs.
Properties
CAS No. |
6651-26-9 |
---|---|
Molecular Formula |
C72H147Al |
Molecular Weight |
1039.9 g/mol |
IUPAC Name |
tri(tetracosyl)alumane |
InChI |
InChI=1S/3C24H49.Al/c3*1-3-5-7-9-11-13-15-17-19-21-23-24-22-20-18-16-14-12-10-8-6-4-2;/h3*1,3-24H2,2H3; |
InChI Key |
MKWOCFYVIMOMNG-UHFFFAOYSA-N |
Canonical SMILES |
CCCCCCCCCCCCCCCCCCCCCCCC[Al](CCCCCCCCCCCCCCCCCCCCCCCC)CCCCCCCCCCCCCCCCCCCCCCCC |
Origin of Product |
United States |
Disclaimer and Information on In-Vitro Research Products
Please be aware that all articles and product information presented on BenchChem are intended solely for informational purposes. The products available for purchase on BenchChem are specifically designed for in-vitro studies, which are conducted outside of living organisms. In-vitro studies, derived from the Latin term "in glass," involve experiments performed in controlled laboratory settings using cells or tissues. It is important to note that these products are not categorized as medicines or drugs, and they have not received approval from the FDA for the prevention, treatment, or cure of any medical condition, ailment, or disease. We must emphasize that any form of bodily introduction of these products into humans or animals is strictly prohibited by law. It is essential to adhere to these guidelines to ensure compliance with legal and ethical standards in research and experimentation.