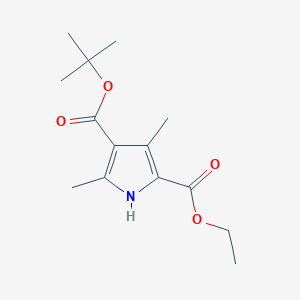
4-Tert-butyl 2-ethyl 3,5-dimethyl-1h-pyrrole-2,4-dicarboxylate
- Click on QUICK INQUIRY to receive a quote from our team of experts.
- With the quality product at a COMPETITIVE price, you can focus more on your research.
Overview
Description
4-Tert-butyl 2-ethyl 3,5-dimethyl-1h-pyrrole-2,4-dicarboxylate is a useful research compound. Its molecular formula is C14H21NO4 and its molecular weight is 267.32 g/mol. The purity is usually 95%.
The exact mass of the compound this compound is unknown and the complexity rating of the compound is unknown. The compound has been submitted to the National Cancer Institute (NCI) for testing and evaluation and the Cancer Chemotherapy National Service Center (NSC) number is 157291. The storage condition is unknown. Please store according to label instructions upon receipt of goods.
BenchChem offers high-quality this compound suitable for many research applications. Different packaging options are available to accommodate customers' requirements. Please inquire for more information about this compound including the price, delivery time, and more detailed information at info@benchchem.com.
Scientific Research Applications
Synthesis and Structural Analysis
4-Tert-butyl 2-ethyl 3,5-dimethyl-1h-pyrrole-2,4-dicarboxylate is involved in various synthesis processes. For instance, Skowronek and Lightner (2003) synthesized a chiral bipyrrole derivative from ethyl 3,5-dimethyl-1H-pyrrole-2-carboxylate, demonstrating its axial chirality and restricted rotation due to tert-butyl groups (Skowronek & Lightner, 2003). Nishio et al. (2011) optimized a novel synthetic method for 4-tert-butyl 2-ethyl 3-amino-1-benzyl-5-dialkylamino-1H-pyrrole-2,4-dicarboxylate derivatives, highlighting the method's selectivity controlled by lithium coordination and steric hindrance (Nishio, Uchiyama, Kito, & Nakahira, 2011).
Chemical Properties and Analysis
Research by Singh et al. (2013) on ethyl-4-[(2,4-dinitrophenyl)-hydrazonomethyl]-3,5-dimethyl-1H-pyrrole-2-carboxylate revealed its properties through quantum chemical calculations, highlighting its potential as a strong electrophile (Singh, Kumar, Tiwari, Rawat, & Manohar, 2013). Ren (2008) synthesized sunitinib malate using 2-tert-butyl-4-ethyl-3,5-dimethyl-1H-pyrrole-2,4-dicarboxylate in a multi-step process, illustrating its application in antitumor agents (Ren, 2008).
Molecular Structure and Hydrogen-Bonding
Senge and Smith (2005) studied the hydrogen-bonding patterns in derivatives of 2,4-dimethylpyrrole, which includes compounds similar to this compound (Senge & Smith, 2005). This research contributes to understanding the molecular structures and interactions of such compounds.
Applications in Organic Syntheses
Various studies have explored the role of similar pyrrole derivatives in organic syntheses. Balaban et al. (2004) synthesized a weak nucleophilic base from a derivative of 3,5-dimethylpyrrole, demonstrating the Janus group effect (Balaban, Ghiviriga, Czerwinski, De, & Faust, 2004). Singh, Rawat, and Sahu (2014) conducted a mixed experimental and DFT study on a pyrrole-containing chalcone derived from ethyl 4-acetyl-3,5-dimethyl-1H-pyrrole-2-carboxylate, exploring its potential as a non-linear optical material (Singh, Rawat, & Sahu, 2014).
Dimer Formation and Spectroscopy Analysis
Research by Singh et al. (2013) on ethyl-4-[(benzoyl)-hydrazonomethyl]-3,5-dimethyl-1H-pyrrole-2-carboxylate revealed multiple interaction formations through spectroscopy and quantum chemical approaches, adding to the understanding of dimer formation in such compounds (Singh, Kumar, Tiwari, & Rawat, 2013).
Safety and Hazards
The safety and hazards associated with “4-Tert-butyl 2-ethyl 3,5-dimethyl-1h-pyrrole-2,4-dicarboxylate” are not fully documented. Sigma-Aldrich provides this product to early discovery researchers as part of a collection of rare and unique chemicals . Buyers are responsible for confirming product identity and/or purity .
Mechanism of Action
Target of Action
This compound is part of a collection of rare and unique chemicals provided for early discovery researchers
Biochemical Pathways
The biochemical pathways affected by 4-Tert-butyl 2-ethyl 3,5-dimethyl-1h-pyrrole-2,4-dicarboxylate are currently unknown . As research progresses, it is expected that the affected pathways and their downstream effects will be elucidated.
Action Environment
The influence of environmental factors on the action, efficacy, and stability of this compound is an area that requires further investigation . Factors such as pH, temperature, and the presence of other molecules could potentially affect the compound’s action.
Biochemical Analysis
Biochemical Properties
4-Tert-butyl 2-ethyl 3,5-dimethyl-1h-pyrrole-2,4-dicarboxylate plays a significant role in biochemical reactions. It interacts with various enzymes, proteins, and other biomolecules. For instance, it has been observed to interact with cytochrome P450 enzymes, which are crucial for the metabolism of many substances in the body . The nature of these interactions often involves the binding of the compound to the active site of the enzyme, potentially inhibiting or modifying its activity.
Cellular Effects
The effects of this compound on cellular processes are profound. It influences cell function by affecting cell signaling pathways, gene expression, and cellular metabolism. For example, it has been shown to modulate the expression of certain genes involved in oxidative stress responses . Additionally, it can alter cellular metabolism by interacting with metabolic enzymes, leading to changes in the levels of various metabolites.
Molecular Mechanism
At the molecular level, this compound exerts its effects through several mechanisms. It binds to specific biomolecules, such as enzymes, and can either inhibit or activate their function. This binding often involves interactions with the enzyme’s active site, leading to changes in the enzyme’s activity. Furthermore, the compound can influence gene expression by interacting with transcription factors or other regulatory proteins .
Temporal Effects in Laboratory Settings
In laboratory settings, the effects of this compound can change over time. The compound’s stability and degradation are important factors to consider. Studies have shown that it remains stable under certain conditions but can degrade over time, leading to changes in its effectiveness . Long-term exposure to the compound in in vitro or in vivo studies has revealed potential impacts on cellular function, including alterations in cell growth and viability.
Dosage Effects in Animal Models
The effects of this compound vary with different dosages in animal models. At lower doses, the compound may exhibit beneficial effects, such as enhancing metabolic processes or protecting against oxidative stress . At higher doses, it can have toxic or adverse effects, including cellular damage and disruption of normal physiological functions. Threshold effects have been observed, indicating that there is a specific dosage range within which the compound is effective without being harmful.
Metabolic Pathways
This compound is involved in several metabolic pathways. It interacts with enzymes such as cytochrome P450, which play a key role in its metabolism . These interactions can affect metabolic flux and the levels of various metabolites. The compound’s metabolism may also involve conjugation reactions, where it is combined with other molecules to form more water-soluble compounds that can be excreted from the body.
Transport and Distribution
Within cells and tissues, this compound is transported and distributed through various mechanisms. It may interact with transporters or binding proteins that facilitate its movement across cell membranes . The compound’s localization and accumulation within specific tissues can influence its overall activity and effectiveness.
Subcellular Localization
The subcellular localization of this compound is crucial for its activity. It may be directed to specific compartments or organelles within the cell through targeting signals or post-translational modifications . This localization can affect the compound’s function, as it may interact with different biomolecules depending on its location within the cell.
Properties
IUPAC Name |
4-O-tert-butyl 2-O-ethyl 3,5-dimethyl-1H-pyrrole-2,4-dicarboxylate |
Source
|
---|---|---|
Source | PubChem | |
URL | https://pubchem.ncbi.nlm.nih.gov | |
Description | Data deposited in or computed by PubChem | |
InChI |
InChI=1S/C14H21NO4/c1-7-18-13(17)11-8(2)10(9(3)15-11)12(16)19-14(4,5)6/h15H,7H2,1-6H3 |
Source
|
Source | PubChem | |
URL | https://pubchem.ncbi.nlm.nih.gov | |
Description | Data deposited in or computed by PubChem | |
InChI Key |
XTQZSZZJWWUQON-UHFFFAOYSA-N |
Source
|
Source | PubChem | |
URL | https://pubchem.ncbi.nlm.nih.gov | |
Description | Data deposited in or computed by PubChem | |
Canonical SMILES |
CCOC(=O)C1=C(C(=C(N1)C)C(=O)OC(C)(C)C)C |
Source
|
Source | PubChem | |
URL | https://pubchem.ncbi.nlm.nih.gov | |
Description | Data deposited in or computed by PubChem | |
Molecular Formula |
C14H21NO4 |
Source
|
Source | PubChem | |
URL | https://pubchem.ncbi.nlm.nih.gov | |
Description | Data deposited in or computed by PubChem | |
DSSTOX Substance ID |
DTXSID70303191 |
Source
|
Record name | 4-tert-butyl 2-ethyl 3,5-dimethyl-1h-pyrrole-2,4-dicarboxylate | |
Source | EPA DSSTox | |
URL | https://comptox.epa.gov/dashboard/DTXSID70303191 | |
Description | DSSTox provides a high quality public chemistry resource for supporting improved predictive toxicology. | |
Molecular Weight |
267.32 g/mol |
Source
|
Source | PubChem | |
URL | https://pubchem.ncbi.nlm.nih.gov | |
Description | Data deposited in or computed by PubChem | |
CAS No. |
361380-77-0 |
Source
|
Record name | 4-tert-butyl 2-ethyl 3,5-dimethyl-1h-pyrrole-2,4-dicarboxylate | |
Source | EPA DSSTox | |
URL | https://comptox.epa.gov/dashboard/DTXSID70303191 | |
Description | DSSTox provides a high quality public chemistry resource for supporting improved predictive toxicology. | |
Disclaimer and Information on In-Vitro Research Products
Please be aware that all articles and product information presented on BenchChem are intended solely for informational purposes. The products available for purchase on BenchChem are specifically designed for in-vitro studies, which are conducted outside of living organisms. In-vitro studies, derived from the Latin term "in glass," involve experiments performed in controlled laboratory settings using cells or tissues. It is important to note that these products are not categorized as medicines or drugs, and they have not received approval from the FDA for the prevention, treatment, or cure of any medical condition, ailment, or disease. We must emphasize that any form of bodily introduction of these products into humans or animals is strictly prohibited by law. It is essential to adhere to these guidelines to ensure compliance with legal and ethical standards in research and experimentation.