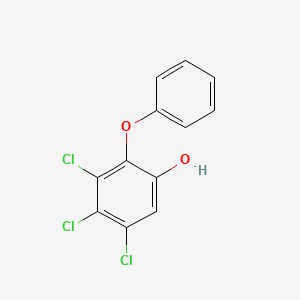
Phenoxyphenol trichloro deriv.
- Click on QUICK INQUIRY to receive a quote from our team of experts.
- With the quality product at a COMPETITIVE price, you can focus more on your research.
Overview
Description
Preparation Methods
Synthetic Routes and Reaction Conditions
The synthesis of phenoxyphenol trichloro derivative typically involves the reaction of 2,4-dichlorophenol with 4-chlorophenol in the presence of a base, such as potassium hydroxide, under reflux conditions . The reaction proceeds through a nucleophilic aromatic substitution mechanism, where the hydroxyl group of 4-chlorophenol attacks the electrophilic carbon of 2,4-dichlorophenol, resulting in the formation of the trichloro derivative.
Industrial Production Methods
Industrial production of phenoxyphenol trichloro derivative often employs continuous flow reactors to ensure efficient mixing and heat transfer. The reaction mixture is typically heated to around 80°C, and the product is isolated through distillation and recrystallization processes to achieve high purity .
Chemical Reactions Analysis
Types of Reactions
Phenoxyphenol trichloro derivative undergoes various chemical reactions, including:
Oxidation: The compound can be oxidized to form quinones, which are important intermediates in organic synthesis.
Substitution: Electrophilic aromatic substitution reactions are common, where the chlorine atoms can be replaced by other substituents.
Common Reagents and Conditions
Oxidation: Common oxidizing agents include potassium permanganate and chromium trioxide.
Reduction: Reducing agents such as sodium borohydride and lithium aluminum hydride are often used.
Substitution: Reagents like bromine and nitric acid are used for halogenation and nitration reactions, respectively.
Major Products Formed
Oxidation: Quinones
Reduction: Hydroquinones
Substitution: Various substituted phenols depending on the reagents used.
Scientific Research Applications
Phenoxyphenol trichloro derivative has a wide range of applications in scientific research:
Mechanism of Action
The antibacterial action of phenoxyphenol trichloro derivative involves the disruption of bacterial cell membranes and inhibition of fatty acid synthesis. The compound targets enoyl-acyl carrier protein reductase, an essential enzyme in the fatty acid synthesis pathway, leading to the inhibition of bacterial growth and eventual cell death .
Comparison with Similar Compounds
Similar Compounds
Triclocarban: Another halogenated phenol with similar antibacterial properties.
Phenoxyethanol: Used as a preservative and has antimicrobial properties.
4-Phenoxyphenol: Known for its porous molecular structure and applications in material science.
Uniqueness
Phenoxyphenol trichloro derivative stands out due to its high efficacy against a broad spectrum of bacteria and its stability in various formulations. Its ability to disrupt bacterial cell membranes and inhibit fatty acid synthesis makes it a valuable compound in both medical and industrial applications .
Properties
CAS No. |
51013-27-5 |
---|---|
Molecular Formula |
C12H7Cl3O2 |
Molecular Weight |
289.5 g/mol |
IUPAC Name |
3,4,5-trichloro-2-phenoxyphenol |
InChI |
InChI=1S/C12H7Cl3O2/c13-8-6-9(16)12(11(15)10(8)14)17-7-4-2-1-3-5-7/h1-6,16H |
InChI Key |
AQBVQIIKUDISJV-UHFFFAOYSA-N |
Canonical SMILES |
C1=CC=C(C=C1)OC2=C(C(=C(C=C2O)Cl)Cl)Cl |
Origin of Product |
United States |
Disclaimer and Information on In-Vitro Research Products
Please be aware that all articles and product information presented on BenchChem are intended solely for informational purposes. The products available for purchase on BenchChem are specifically designed for in-vitro studies, which are conducted outside of living organisms. In-vitro studies, derived from the Latin term "in glass," involve experiments performed in controlled laboratory settings using cells or tissues. It is important to note that these products are not categorized as medicines or drugs, and they have not received approval from the FDA for the prevention, treatment, or cure of any medical condition, ailment, or disease. We must emphasize that any form of bodily introduction of these products into humans or animals is strictly prohibited by law. It is essential to adhere to these guidelines to ensure compliance with legal and ethical standards in research and experimentation.