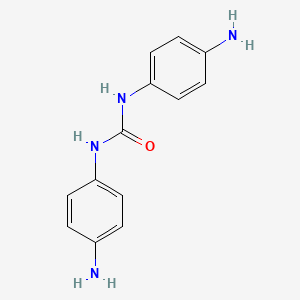
1,3-Bis(4-Aminophenyl)urea
Overview
Description
Mechanism of Action
Target of Action
The primary target of 1,3-Bis(4-Aminophenyl)urea is the Mitochondrial membrane protein voltage-dependent anion channel 1 (VDAC1) . VDAC1 plays a crucial role in controlling the passage of metabolites between the mitochondria and the cytoplasm, and it is also involved in mitochondria-mediated apoptosis .
Mode of Action
This compound interacts directly with VDAC1 and prevents its oligomerization . This interaction inhibits the formation of the VDAC1 channel, thereby modulating the process of apoptosis .
Biochemical Pathways
The inhibition of VDAC1 oligomerization by this compound affects the apoptosis pathway. Apoptosis, or programmed cell death, is a crucial process in cellular homeostasis and development. By modulating VDAC1, this compound can influence the balance between cell survival and death .
Pharmacokinetics
It’s known that the compound is a solid powder that is insoluble in water but soluble in organic solvents such as dmso . These properties may affect its absorption, distribution, metabolism, and excretion (ADME), and thus its bioavailability.
Result of Action
The result of the action of this compound is the modulation of apoptosis. By preventing the oligomerization of VDAC1, it can inhibit apoptosis, potentially promoting cell survival .
Action Environment
The action of this compound can be influenced by various environmental factors. For instance, it should be stored at -20°C and in a dark place to maintain its stability .
Biochemical Analysis
Biochemical Properties
1,3-Bis(4-Aminophenyl)urea plays a significant role in biochemical reactions, particularly in the inhibition of apoptosis. It interacts directly with the mitochondrial membrane protein voltage-dependent anion channel 1 (VDAC1), preventing its oligomerization and thereby inhibiting apoptosis . This interaction is crucial as VDAC1 oligomerization is a prime target for agents designed to modulate apoptosis.
Cellular Effects
This compound has notable effects on various types of cells and cellular processes. By inhibiting VDAC1 oligomerization, it prevents the initiation of apoptosis, thereby influencing cell survival . This compound also affects cell signaling pathways, gene expression, and cellular metabolism by modulating the activity of VDAC1, which is involved in the regulation of mitochondrial function and energy production.
Molecular Mechanism
At the molecular level, this compound exerts its effects by binding to VDAC1 and preventing its oligomerization . This inhibition of VDAC1 oligomerization is critical in preventing the release of apoptogenic factors from the mitochondria, thereby inhibiting the apoptotic pathway. Additionally, this compound may influence gene expression by modulating the activity of transcription factors involved in apoptosis regulation.
Temporal Effects in Laboratory Settings
In laboratory settings, the effects of this compound have been observed to change over time. The compound is relatively stable under standard storage conditions, but its activity may degrade over extended periods . Long-term studies have shown that continuous exposure to this compound can lead to sustained inhibition of apoptosis, with minimal degradation observed in vitro .
Dosage Effects in Animal Models
The effects of this compound vary with different dosages in animal models. At lower doses, the compound effectively inhibits apoptosis without causing significant toxicity . At higher doses, it may exhibit toxic effects, including potential disruption of mitochondrial function and induction of oxidative stress . These dosage-dependent effects highlight the importance of optimizing the dosage to achieve the desired therapeutic outcomes while minimizing adverse effects.
Metabolic Pathways
This compound is involved in metabolic pathways related to apoptosis regulation. It interacts with enzymes and cofactors that modulate the activity of VDAC1 and other proteins involved in the apoptotic pathway . This compound may also affect metabolic flux and metabolite levels by influencing mitochondrial function and energy production.
Transport and Distribution
Within cells and tissues, this compound is transported and distributed through interactions with specific transporters and binding proteins . These interactions facilitate its localization to the mitochondria, where it exerts its inhibitory effects on VDAC1 oligomerization. The compound’s distribution is crucial for its activity, as it needs to reach the mitochondria to effectively inhibit apoptosis.
Subcellular Localization
This compound is primarily localized to the mitochondria, where it interacts with VDAC1 . This subcellular localization is essential for its function, as it allows the compound to directly inhibit VDAC1 oligomerization and prevent the release of apoptogenic factors. The targeting of this compound to the mitochondria may be facilitated by specific targeting signals or post-translational modifications that direct it to this organelle.
Preparation Methods
Synthetic Routes and Reaction Conditions
The synthesis of NSC15364 involves the reaction of 4-nitroaniline with phosgene to form 1,3-bis(4-nitrophenyl)urea, which is then reduced to 1,3-bis(4-aminophenyl)urea using a reducing agent such as iron powder in the presence of hydrochloric acid .
Industrial Production Methods
While specific industrial production methods for NSC15364 are not widely documented, the general approach involves scaling up the laboratory synthesis process. This includes optimizing reaction conditions, such as temperature, pressure, and solvent choice, to ensure high yield and purity .
Chemical Reactions Analysis
Types of Reactions
NSC15364 undergoes several types of chemical reactions, including:
Reduction: The nitro groups in the precursor compound are reduced to amino groups.
Substitution: The formation of the urea linkage involves a substitution reaction where phosgene reacts with 4-nitroaniline.
Common Reagents and Conditions
Reduction: Iron powder and hydrochloric acid are commonly used for the reduction of nitro groups to amino groups.
Substitution: Phosgene is used in the formation of the urea linkage.
Major Products
Scientific Research Applications
NSC15364 has a wide range of scientific research applications, including:
Comparison with Similar Compounds
Similar Compounds
- MeOSuc-Ala-Ala-Pro-Val-pNA
- BDP 558/568 amine
- AC-green
- Naphthalene-1,2-dicarbaldehyde
- Rhodamine 800
- Vari Fluor 532 SE
- Coumarin-PEG2-SCO
- Acridine Orange hydrochloride
- N-(7-Nitrobenzofurazan-4-yl)phallacidin
- WSP-5
- Chromoionophore XIII
- FRETS-VWF73
- Biotin-11-UTP
- BODIPY FL SSE
- 3-HTC
- C.I. Pigment orange 34
- 1,2-Diamino-3,4-ethylenedioxybenzene
- TO-PRO-3 iodide
- Sulfo-CY-5.5 NHS ester
- tripotassium ATTO 647 NHS ester
- Quinoline yellow 2SF
- DiSulfo-ICG-azide disodium
- BODIPY 505/515
- 3-Acetylumbelliferyl β-D-Glucopyranoside
- Ethyl 8’-apo-caroten-8’-oate
- Ac-DNLD-AMC
- Mucicarmine
- Maackia Amurensis Lectin II
- Disperse Red 278
- Solvent blue 97 .
Uniqueness
NSC15364 is unique due to its specific inhibition of VDAC1 oligomerization, which is a critical step in the apoptotic process . This makes it a valuable tool for studying apoptosis and developing therapeutic agents targeting diseases where apoptosis is dysregulated .
Properties
IUPAC Name |
1,3-bis(4-aminophenyl)urea | |
---|---|---|
Source | PubChem | |
URL | https://pubchem.ncbi.nlm.nih.gov | |
Description | Data deposited in or computed by PubChem | |
InChI |
InChI=1S/C13H14N4O/c14-9-1-5-11(6-2-9)16-13(18)17-12-7-3-10(15)4-8-12/h1-8H,14-15H2,(H2,16,17,18) | |
Source | PubChem | |
URL | https://pubchem.ncbi.nlm.nih.gov | |
Description | Data deposited in or computed by PubChem | |
InChI Key |
MAPWYRGGJSHAAU-UHFFFAOYSA-N | |
Source | PubChem | |
URL | https://pubchem.ncbi.nlm.nih.gov | |
Description | Data deposited in or computed by PubChem | |
Canonical SMILES |
C1=CC(=CC=C1N)NC(=O)NC2=CC=C(C=C2)N | |
Source | PubChem | |
URL | https://pubchem.ncbi.nlm.nih.gov | |
Description | Data deposited in or computed by PubChem | |
Molecular Formula |
C13H14N4O | |
Source | PubChem | |
URL | https://pubchem.ncbi.nlm.nih.gov | |
Description | Data deposited in or computed by PubChem | |
DSSTOX Substance ID |
DTXSID70280079 | |
Record name | 1,3-Bis(4-Aminophenyl)urea | |
Source | EPA DSSTox | |
URL | https://comptox.epa.gov/dashboard/DTXSID70280079 | |
Description | DSSTox provides a high quality public chemistry resource for supporting improved predictive toxicology. | |
Molecular Weight |
242.28 g/mol | |
Source | PubChem | |
URL | https://pubchem.ncbi.nlm.nih.gov | |
Description | Data deposited in or computed by PubChem | |
CAS No. |
4550-72-5 | |
Record name | 1,3-Bis(4-Aminophenyl)urea | |
Source | DTP/NCI | |
URL | https://dtp.cancer.gov/dtpstandard/servlet/dwindex?searchtype=NSC&outputformat=html&searchlist=15364 | |
Description | The NCI Development Therapeutics Program (DTP) provides services and resources to the academic and private-sector research communities worldwide to facilitate the discovery and development of new cancer therapeutic agents. | |
Explanation | Unless otherwise indicated, all text within NCI products is free of copyright and may be reused without our permission. Credit the National Cancer Institute as the source. | |
Record name | 1,3-Bis(4-Aminophenyl)urea | |
Source | EPA DSSTox | |
URL | https://comptox.epa.gov/dashboard/DTXSID70280079 | |
Description | DSSTox provides a high quality public chemistry resource for supporting improved predictive toxicology. | |
Synthesis routes and methods
Procedure details
Retrosynthesis Analysis
AI-Powered Synthesis Planning: Our tool employs the Template_relevance Pistachio, Template_relevance Bkms_metabolic, Template_relevance Pistachio_ringbreaker, Template_relevance Reaxys, Template_relevance Reaxys_biocatalysis model, leveraging a vast database of chemical reactions to predict feasible synthetic routes.
One-Step Synthesis Focus: Specifically designed for one-step synthesis, it provides concise and direct routes for your target compounds, streamlining the synthesis process.
Accurate Predictions: Utilizing the extensive PISTACHIO, BKMS_METABOLIC, PISTACHIO_RINGBREAKER, REAXYS, REAXYS_BIOCATALYSIS database, our tool offers high-accuracy predictions, reflecting the latest in chemical research and data.
Strategy Settings
Precursor scoring | Relevance Heuristic |
---|---|
Min. plausibility | 0.01 |
Model | Template_relevance |
Template Set | Pistachio/Bkms_metabolic/Pistachio_ringbreaker/Reaxys/Reaxys_biocatalysis |
Top-N result to add to graph | 6 |
Feasible Synthetic Routes
Q1: What makes 1,3-Bis(4-Aminophenyl)urea suitable for creating porous organic polymers?
A: this compound possesses a unique structure that makes it ideal for constructing porous organic polymers (POPs). The molecule features two amine groups (-NH2) positioned at opposite ends, allowing it to react with suitable linkers and form extended, repeating networks. [, ] These networks create the porous structure characteristic of POPs. Additionally, the urea group (-NH-CO-NH-) within the molecule contributes to the material's stability and can participate in hydrogen bonding, potentially influencing guest molecule interactions within the pores. [, ]
Q2: How does incorporating Palladium into a this compound-based porous organic polymer affect its performance in electrochemical sensing?
A: Research has shown that incorporating Palladium nanoparticles into a this compound-based porous organic polymer (Pd@TU-POP) significantly enhances its ability to detect rutin electrochemically. [] The Palladium nanoparticles act as electrocatalysts, facilitating the oxidation of rutin and reducing the overpotential required for detection. This translates to a more sensitive and efficient sensor. Furthermore, the porous structure of the polymer provides excellent structural support for the Palladium nanoparticles, ensuring their even distribution and stability, which contributes to the sensor's overall performance and lifespan. []
Q3: Can this compound-based porous organic polymers be used for size-selective catalysis?
A: Yes, research indicates that a this compound-based covalent organic framework (COF), a specific type of porous organic polymer, named TpMU, exhibits size-selective catalytic activity. [] This COF, synthesized by reacting this compound with 1,3,5-Triformylphloroglucinol, displays high crystallinity and a well-defined pore structure, confirmed through techniques like Powder X-ray Diffraction and Nitrogen adsorption isotherm analysis. [] This specific pore structure allows TpMU to selectively catalyze reactions based on the size of the reactant molecules, making it a promising material for applications requiring precise control over reaction products. []
Disclaimer and Information on In-Vitro Research Products
Please be aware that all articles and product information presented on BenchChem are intended solely for informational purposes. The products available for purchase on BenchChem are specifically designed for in-vitro studies, which are conducted outside of living organisms. In-vitro studies, derived from the Latin term "in glass," involve experiments performed in controlled laboratory settings using cells or tissues. It is important to note that these products are not categorized as medicines or drugs, and they have not received approval from the FDA for the prevention, treatment, or cure of any medical condition, ailment, or disease. We must emphasize that any form of bodily introduction of these products into humans or animals is strictly prohibited by law. It is essential to adhere to these guidelines to ensure compliance with legal and ethical standards in research and experimentation.