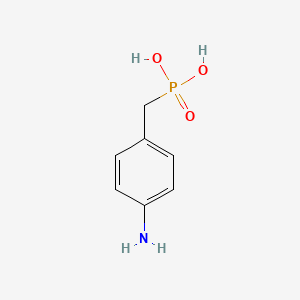
4-Aminobenzylphosphonic acid
Overview
Description
4-Aminobenzylphosphonic acid is a useful research compound. Its molecular formula is C7H10NO3P and its molecular weight is 187.13 g/mol. The purity is usually 95%.
The exact mass of the compound this compound is unknown and the complexity rating of the compound is unknown. The compound has been submitted to the National Cancer Institute (NCI) for testing and evaluation and the Cancer Chemotherapy National Service Center (NSC) number is 12117. The United Nations designated GHS hazard class pictogram is Irritant, and the GHS signal word is WarningThe storage condition is unknown. Please store according to label instructions upon receipt of goods.
BenchChem offers high-quality this compound suitable for many research applications. Different packaging options are available to accommodate customers' requirements. Please inquire for more information about this compound including the price, delivery time, and more detailed information at info@benchchem.com.
Mechanism of Action
Target of Action
4-Aminobenzylphosphonic acid is primarily an inhibitor of alkaline phosphatase . Alkaline phosphatase is an enzyme that plays a crucial role in several biochemical processes, including phosphate metabolism and signal transduction.
Mode of Action
The compound interacts with its target, alkaline phosphatase, by binding to it and inhibiting its function
Result of Action
The molecular and cellular effects of this compound’s action primarily involve the inhibition of alkaline phosphatase activity . This can lead to alterations in phosphate metabolism and signal transduction, potentially affecting a variety of cellular functions.
Biochemical Analysis
Biochemical Properties
4-Aminobenzylphosphonic acid plays a significant role in biochemical reactions, particularly as an inhibitor of alkaline phosphatase . This enzyme is crucial in dephosphorylation processes, and its inhibition by this compound can affect various metabolic pathways. The compound interacts with the active site of alkaline phosphatase, preventing the enzyme from catalyzing the removal of phosphate groups from substrates . This interaction is essential for studying the enzyme’s function and regulation in different biological contexts.
Cellular Effects
This compound influences various cellular processes by inhibiting alkaline phosphatase activity. This inhibition can lead to changes in cell signaling pathways, gene expression, and cellular metabolism . For instance, the compound’s effect on dephosphorylation processes can alter the phosphorylation state of proteins involved in signaling pathways, thereby affecting cell function and behavior. Additionally, this compound may impact cellular metabolism by modulating the activity of enzymes involved in metabolic pathways.
Molecular Mechanism
The molecular mechanism of this compound involves its binding to the active site of alkaline phosphatase, where it acts as a competitive inhibitor . By occupying the active site, the compound prevents the enzyme from interacting with its natural substrates, thereby inhibiting its catalytic activity. This inhibition can lead to downstream effects on various biochemical pathways, as the dephosphorylation of key proteins is disrupted. Additionally, this compound may influence gene expression by affecting the phosphorylation state of transcription factors and other regulatory proteins.
Temporal Effects in Laboratory Settings
In laboratory settings, the effects of this compound can change over time due to its stability and degradation. The compound is generally stable under standard storage conditions, but its activity may decrease over extended periods . Long-term studies have shown that the inhibition of alkaline phosphatase by this compound can lead to sustained changes in cellular function, including alterations in gene expression and metabolic activity. These effects are particularly evident in in vitro studies, where the compound’s stability and activity can be closely monitored.
Dosage Effects in Animal Models
The effects of this compound in animal models vary with different dosages. At low doses, the compound effectively inhibits alkaline phosphatase without causing significant toxicity . At higher doses, this compound may exhibit toxic effects, including adverse impacts on liver and kidney function. These threshold effects are crucial for determining the safe and effective dosage range for potential therapeutic applications. Studies in animal models have also highlighted the importance of dosage in modulating the compound’s biochemical and cellular effects.
Metabolic Pathways
This compound is involved in metabolic pathways related to phosphate metabolism . By inhibiting alkaline phosphatase, the compound affects the dephosphorylation of various substrates, including nucleotides, proteins, and small molecules. This inhibition can lead to changes in metabolic flux and metabolite levels, as the balance between phosphorylated and dephosphorylated states is disrupted. Additionally, this compound may interact with other enzymes and cofactors involved in phosphate metabolism, further influencing metabolic pathways.
Transport and Distribution
Within cells and tissues, this compound is transported and distributed through various mechanisms . The compound may interact with transporters and binding proteins that facilitate its uptake and localization. Once inside the cell, this compound can accumulate in specific compartments, such as the cytoplasm and nucleus, where it exerts its inhibitory effects on alkaline phosphatase. The distribution of the compound within tissues can also affect its overall activity and efficacy in different biological contexts.
Subcellular Localization
The subcellular localization of this compound is crucial for its activity and function . The compound is primarily localized in the cytoplasm and nucleus, where it interacts with alkaline phosphatase and other target proteins. This localization is facilitated by specific targeting signals and post-translational modifications that direct this compound to its sites of action. The compound’s activity may be influenced by its subcellular distribution, as the concentration and availability of target proteins can vary between different cellular compartments.
Properties
IUPAC Name |
(4-aminophenyl)methylphosphonic acid | |
---|---|---|
Source | PubChem | |
URL | https://pubchem.ncbi.nlm.nih.gov | |
Description | Data deposited in or computed by PubChem | |
InChI |
InChI=1S/C7H10NO3P/c8-7-3-1-6(2-4-7)5-12(9,10)11/h1-4H,5,8H2,(H2,9,10,11) | |
Source | PubChem | |
URL | https://pubchem.ncbi.nlm.nih.gov | |
Description | Data deposited in or computed by PubChem | |
InChI Key |
NEKHKXMBGWNTOO-UHFFFAOYSA-N | |
Source | PubChem | |
URL | https://pubchem.ncbi.nlm.nih.gov | |
Description | Data deposited in or computed by PubChem | |
Canonical SMILES |
C1=CC(=CC=C1CP(=O)(O)O)N | |
Source | PubChem | |
URL | https://pubchem.ncbi.nlm.nih.gov | |
Description | Data deposited in or computed by PubChem | |
Molecular Formula |
C7H10NO3P | |
Source | PubChem | |
URL | https://pubchem.ncbi.nlm.nih.gov | |
Description | Data deposited in or computed by PubChem | |
DSSTOX Substance ID |
DTXSID40279293 | |
Record name | 4-Aminobenzylphosphonic acid | |
Source | EPA DSSTox | |
URL | https://comptox.epa.gov/dashboard/DTXSID40279293 | |
Description | DSSTox provides a high quality public chemistry resource for supporting improved predictive toxicology. | |
Molecular Weight |
187.13 g/mol | |
Source | PubChem | |
URL | https://pubchem.ncbi.nlm.nih.gov | |
Description | Data deposited in or computed by PubChem | |
CAS No. |
5424-27-1 | |
Record name | 5424-27-1 | |
Source | DTP/NCI | |
URL | https://dtp.cancer.gov/dtpstandard/servlet/dwindex?searchtype=NSC&outputformat=html&searchlist=12117 | |
Description | The NCI Development Therapeutics Program (DTP) provides services and resources to the academic and private-sector research communities worldwide to facilitate the discovery and development of new cancer therapeutic agents. | |
Explanation | Unless otherwise indicated, all text within NCI products is free of copyright and may be reused without our permission. Credit the National Cancer Institute as the source. | |
Record name | 4-Aminobenzylphosphonic acid | |
Source | EPA DSSTox | |
URL | https://comptox.epa.gov/dashboard/DTXSID40279293 | |
Description | DSSTox provides a high quality public chemistry resource for supporting improved predictive toxicology. | |
Record name | (4-Aminobenzyl)phosphonic Acid | |
Source | European Chemicals Agency (ECHA) | |
URL | https://echa.europa.eu/information-on-chemicals | |
Description | The European Chemicals Agency (ECHA) is an agency of the European Union which is the driving force among regulatory authorities in implementing the EU's groundbreaking chemicals legislation for the benefit of human health and the environment as well as for innovation and competitiveness. | |
Explanation | Use of the information, documents and data from the ECHA website is subject to the terms and conditions of this Legal Notice, and subject to other binding limitations provided for under applicable law, the information, documents and data made available on the ECHA website may be reproduced, distributed and/or used, totally or in part, for non-commercial purposes provided that ECHA is acknowledged as the source: "Source: European Chemicals Agency, http://echa.europa.eu/". Such acknowledgement must be included in each copy of the material. ECHA permits and encourages organisations and individuals to create links to the ECHA website under the following cumulative conditions: Links can only be made to webpages that provide a link to the Legal Notice page. | |
Synthesis routes and methods
Procedure details
Retrosynthesis Analysis
AI-Powered Synthesis Planning: Our tool employs the Template_relevance Pistachio, Template_relevance Bkms_metabolic, Template_relevance Pistachio_ringbreaker, Template_relevance Reaxys, Template_relevance Reaxys_biocatalysis model, leveraging a vast database of chemical reactions to predict feasible synthetic routes.
One-Step Synthesis Focus: Specifically designed for one-step synthesis, it provides concise and direct routes for your target compounds, streamlining the synthesis process.
Accurate Predictions: Utilizing the extensive PISTACHIO, BKMS_METABOLIC, PISTACHIO_RINGBREAKER, REAXYS, REAXYS_BIOCATALYSIS database, our tool offers high-accuracy predictions, reflecting the latest in chemical research and data.
Strategy Settings
Precursor scoring | Relevance Heuristic |
---|---|
Min. plausibility | 0.01 |
Model | Template_relevance |
Template Set | Pistachio/Bkms_metabolic/Pistachio_ringbreaker/Reaxys/Reaxys_biocatalysis |
Top-N result to add to graph | 6 |
Feasible Synthetic Routes
Q1: What are the primary applications of 4-Aminobenzylphosphonic acid in material science?
A: this compound is primarily explored for its ability to modify electrode surfaces. Research demonstrates its use in electrografting stainless steel [] and glassy carbon electrodes [, ]. This modification is particularly interesting for developing electrochemical sensors.
Q2: How does this compound interact with electrode surfaces during modification?
A: While the provided abstracts don't delve into the specific interaction mechanism, research suggests that the electrochemical oxidation of this compound plays a crucial role in its covalent attachment to glassy carbon electrodes []. This likely involves the formation of reactive intermediates that bind to the electrode surface.
Q3: Are there any specific applications for this compound modified electrodes?
A: Yes, research indicates that glassy carbon electrodes modified with this compound show promise for electrochemically sensing paracetamol []. The modification likely enhances the sensitivity and selectivity of the electrode towards paracetamol detection.
Disclaimer and Information on In-Vitro Research Products
Please be aware that all articles and product information presented on BenchChem are intended solely for informational purposes. The products available for purchase on BenchChem are specifically designed for in-vitro studies, which are conducted outside of living organisms. In-vitro studies, derived from the Latin term "in glass," involve experiments performed in controlled laboratory settings using cells or tissues. It is important to note that these products are not categorized as medicines or drugs, and they have not received approval from the FDA for the prevention, treatment, or cure of any medical condition, ailment, or disease. We must emphasize that any form of bodily introduction of these products into humans or animals is strictly prohibited by law. It is essential to adhere to these guidelines to ensure compliance with legal and ethical standards in research and experimentation.