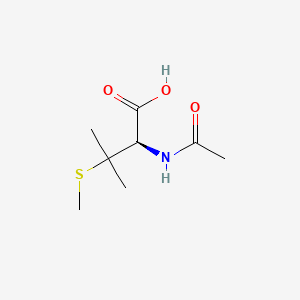
S-Methyl-N-acetylpenicillamine
- Click on QUICK INQUIRY to receive a quote from our team of experts.
- With the quality product at a COMPETITIVE price, you can focus more on your research.
Overview
Description
S-Methyl-N-acetylpenicillamine: is an organosulfur compound with the chemical formula C7H13NO2S It is a derivative of penicillamine, an amino acid known for its chelating properties
Preparation Methods
Synthetic Routes and Reaction Conditions:
Starting Materials: The synthesis of S-Methyl-N-acetylpenicillamine typically begins with N-acetylpenicillamine.
Methylation: The key step involves the methylation of the sulfur atom in N-acetylpenicillamine. This can be achieved using methyl iodide (CH3I) in the presence of a base such as sodium hydroxide (NaOH).
Reaction Conditions: The reaction is usually carried out in an organic solvent like dimethyl sulfoxide (DMSO) at room temperature. The reaction mixture is stirred for several hours to ensure complete methylation.
Industrial Production Methods: Industrial production of this compound follows similar synthetic routes but on a larger scale. The process involves:
Bulk Reactors: Using large-scale reactors to handle the increased volume of reactants.
Purification: Employing techniques such as crystallization or chromatography to purify the final product.
Quality Control: Ensuring the product meets industry standards through rigorous quality control measures.
Chemical Reactions Analysis
Types of Reactions:
Oxidation: S-Methyl-N-acetylpenicillamine can undergo oxidation reactions, forming disulfides or sulfoxides.
Reduction: It can be reduced back to its thiol form using reducing agents like dithiothreitol (DTT).
Substitution: The compound can participate in nucleophilic substitution reactions, where the methyl group can be replaced by other nucleophiles.
Common Reagents and Conditions:
Oxidation: Hydrogen peroxide (H2O2) or other oxidizing agents under mild conditions.
Reduction: Dithiothreitol (DTT) or sodium borohydride (NaBH4) in aqueous or organic solvents.
Substitution: Various nucleophiles such as thiols or amines in the presence of a base.
Major Products:
Oxidation Products: Disulfides or sulfoxides.
Reduction Products: Thiol derivatives.
Substitution Products: Compounds with different functional groups replacing the methyl group.
Scientific Research Applications
Chemistry:
Catalysis: S-Methyl-N-acetylpenicillamine is used as a ligand in catalytic reactions, enhancing the efficiency of metal-catalyzed processes.
Biology:
Enzyme Inhibition: It acts as an inhibitor for certain enzymes, making it useful in studying enzyme mechanisms and functions.
Medicine:
Chelation Therapy: Due to its chelating properties, it is explored for use in chelation therapy to treat heavy metal poisoning.
Industry:
Material Science: The compound is used in the development of new materials with specific properties, such as enhanced conductivity or stability.
Mechanism of Action
Molecular Targets and Pathways: S-Methyl-N-acetylpenicillamine exerts its effects primarily through its sulfur atom, which can interact with metal ions or thiol groups in proteins. This interaction can inhibit enzyme activity or alter protein function, leading to various biological effects. The compound can also release nitric oxide (NO), a signaling molecule involved in vasodilation and other physiological processes.
Comparison with Similar Compounds
S-Nitroso-N-acetylpenicillamine: Similar in structure but contains a nitroso group, making it a nitric oxide donor.
N-Acetylpenicillamine: The parent compound without the methyl group, used primarily for its chelating properties.
S-Methylcysteine: Another sulfur-containing amino acid derivative with similar reactivity.
Uniqueness: S-Methyl-N-acetylpenicillamine is unique due to its specific methylation, which alters its reactivity and potential applications compared to its parent compound and other derivatives. This methylation enhances its stability and makes it a more effective ligand in catalytic processes.
Properties
CAS No. |
34297-29-5 |
---|---|
Molecular Formula |
C8H15NO3S |
Molecular Weight |
205.28 g/mol |
IUPAC Name |
(2R)-2-acetamido-3-methyl-3-methylsulfanylbutanoic acid |
InChI |
InChI=1S/C8H15NO3S/c1-5(10)9-6(7(11)12)8(2,3)13-4/h6H,1-4H3,(H,9,10)(H,11,12)/t6-/m1/s1 |
InChI Key |
CZLUCNVYZJBVPR-ZCFIWIBFSA-N |
Isomeric SMILES |
CC(=O)N[C@H](C(=O)O)C(C)(C)SC |
Canonical SMILES |
CC(=O)NC(C(=O)O)C(C)(C)SC |
Origin of Product |
United States |
Disclaimer and Information on In-Vitro Research Products
Please be aware that all articles and product information presented on BenchChem are intended solely for informational purposes. The products available for purchase on BenchChem are specifically designed for in-vitro studies, which are conducted outside of living organisms. In-vitro studies, derived from the Latin term "in glass," involve experiments performed in controlled laboratory settings using cells or tissues. It is important to note that these products are not categorized as medicines or drugs, and they have not received approval from the FDA for the prevention, treatment, or cure of any medical condition, ailment, or disease. We must emphasize that any form of bodily introduction of these products into humans or animals is strictly prohibited by law. It is essential to adhere to these guidelines to ensure compliance with legal and ethical standards in research and experimentation.