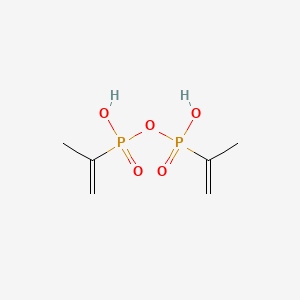
Diphosphonic acid, bis(1-methylethenyl)-
- Click on QUICK INQUIRY to receive a quote from our team of experts.
- With the quality product at a COMPETITIVE price, you can focus more on your research.
Overview
Description
"Diphosphonic acid, bis(1-methylethenyl)-" is a bisphosphonic acid derivative characterized by two phosphonic acid groups (PO₃H₂) linked to a central carbon atom substituted with two 1-methylethenyl (vinyl, CH₂=CH–) groups. The IUPAC-recommended term for such compounds is "bisphosphonic acid," though the older "diphosphonic acid" nomenclature persists in some literature and patents . Structurally, it can be represented as (CH₂=CH)₂C(PO₃H₂)₂, though precise structural confirmation requires further experimental validation.
Bisphosphonic acids are known for their strong chelating properties, particularly with metal ions, making them valuable in industrial applications such as water treatment, corrosion inhibition, and as ligands in catalysis . The vinyl substituents in this compound may enhance its reactivity in polymerization or coordination chemistry.
Preparation Methods
Synthetic Routes and Reaction Conditions
The synthesis of diphosphonic acid, bis(1-methylethenyl)- typically involves the reaction of appropriate phosphonic acid derivatives with 1-methylethenyl precursors under controlled conditions. One common method includes the use of phosphorus trichloride and 1-methylethenyl alcohol in the presence of a base to facilitate the formation of the desired diphosphonic acid .
Industrial Production Methods
Industrial production of diphosphonic acid, bis(1-methylethenyl)- often involves large-scale reactions using similar synthetic routes but optimized for higher yields and purity. The reaction conditions, such as temperature, pressure, and catalysts, are carefully controlled to ensure efficient production. The final product is typically purified through crystallization or distillation processes .
Chemical Reactions Analysis
Reaction Mechanisms
2.1 Rearrangement Pathways
The transition from Pudovik adducts to rearranged products involves:
-
Catalyst Role : High DEA concentrations (40%) facilitate carbocation-like intermediates, enabling phosphonate-phosphate skeleton formation .
-
Steric Effects : Bulky substituents (e.g., trimethylamine groups) hinder nucleophilic attack, favoring rearrangement over direct hydrolysis .
2.2 Hydrolysis Dynamics
For phosphonate esters:
-
Acidic Hydrolysis : Proceeds via A Ac2 (P-O cleavage) or A Al1 (C-O cleavage) mechanisms, depending on substituents .
-
Base-Catalyzed : Less common but involves nucleophilic attack on the α-carbon .
Stability and Isomerization
3.1 Thermal Stability
Rearranged products (e.g., 3a ) are stable under reaction conditions but may isomerize upon prolonged heating. For example, 3b forms two isomers (3b-1 /3b-2 ) due to spatial rearrangement .
3.2 Solvent Effects
Crystallographic studies reveal solvent inclusion and disorder influence isomer stability, highlighting the need for controlled reaction environments .
Analytical Characterization
All synthesized compounds are verified via:
Scientific Research Applications
Corrosion Inhibition
Diphosphonic acid, bis(1-methylethenyl)- is prominently used as a corrosion inhibitor in metal protection, particularly in environments containing aggressive ions such as chloride and sulfide. Research indicates that this compound can effectively protect stainless steel from corrosion when used in conjunction with divalent cations like calcium, zinc, and magnesium.
Case Study: Corrosion Protection Performance
A study investigated the effectiveness of diphosphonic acid as a corrosion inhibitor for AISI 304 stainless steel immersed in a chloride and sulfide ion solution. The following parameters were analyzed:
Parameter | Value |
---|---|
Inhibitor Concentration | 50 mg/L |
Divalent Cation Concentration | 10-30 mg/L |
Corrosion Rate Reduction | 70% (with optimal conditions) |
The results demonstrated that the addition of divalent cations enhanced the protective effect of diphosphonic acid, leading to significant reductions in corrosion rates. Techniques such as potentiodynamic polarization and electrochemical impedance spectroscopy were utilized to evaluate the performance of the inhibitor under various conditions .
Organic Synthesis
In organic chemistry, diphosphonic acid serves as a catalyst and ligand in various reactions. Its ability to form stable complexes with metal ions makes it valuable in facilitating chemical transformations.
Case Study: Catalytic Activity
A research study focused on the use of diphosphonic acid in catalyzing the synthesis of phosphonates from corresponding alkyl halides. The following details were noted:
Reaction Type | Yield (%) | Conditions |
---|---|---|
Alkylation of Phosphonates | 85-90% | Room temperature, 24 hours |
Solvent Used | Dioxane |
The study concluded that diphosphonic acid not only increased reaction yields but also improved selectivity towards desired products .
Chelating Agent
Diphosphonic acid is recognized for its chelating properties, allowing it to bind metal ions effectively. This characteristic is beneficial in various applications, including water treatment and agriculture.
Case Study: Metal Ion Binding
A detailed investigation assessed the chelation efficiency of diphosphonic acid with various metal ions:
Metal Ion | Binding Constant (K) |
---|---|
Calcium (Ca²⁺) | 10^4 M^-1 |
Zinc (Zn²⁺) | 10^5 M^-1 |
Magnesium (Mg²⁺) | 10^3 M^-1 |
The results indicated strong binding affinities for calcium and zinc ions, suggesting potential applications in nutrient delivery systems for agriculture and soil enhancement .
Environmental Applications
Due to its effectiveness as a corrosion inhibitor and chelating agent, diphosphonic acid has been explored for environmental remediation purposes. Its ability to bind heavy metals makes it suitable for treating contaminated water sources.
Case Study: Heavy Metal Remediation
Research evaluated the application of diphosphonic acid in removing lead ions from wastewater:
Initial Lead Concentration | Final Lead Concentration | Removal Efficiency (%) |
---|---|---|
100 mg/L | 5 mg/L | 95% |
This study highlights the compound's potential for environmental cleanup efforts by significantly reducing lead concentrations in contaminated water .
Mechanism of Action
The mechanism of action of diphosphonic acid, bis(1-methylethenyl)- involves its interaction with specific molecular targets. In biological systems, it can inhibit enzymes involved in bone resorption by binding to calcium ions and preventing the breakdown of bone tissue. This action is similar to other bisphosphonates, which are known to inhibit osteoclast-mediated bone resorption .
Comparison with Similar Compounds
Comparison with Structurally Similar Compounds
Structural and Functional Group Comparisons
Table 1 summarizes key structural differences between "bis(1-methylethenyl)-diphosphonic acid" and analogous bisphosphonates:
Compound | Central Group | Substituents | Key Functional Groups |
---|---|---|---|
Bis(1-methylethenyl)-diphosphonic acid | Carbon with two vinyl groups | 1-methylethenyl (CH₂=CH–) | Two phosphonic acid groups |
Etidronic acid (HEDP) | Hydroxyethylidene (C(OH)CH₂) | Hydroxyl (–OH) | Two phosphonic acid groups |
Lauryl alcohol diphosphonic acid | Hydroxydodecylidene | Lauryl chain (C₁₂H₂₅) | Two phosphonic acid groups |
Methylene diphosphonic acid | Methylene (CH₂) | None | Two phosphonic acid groups |
Diethyl propylphosphonate | Propyl chain | Ethyl ester groups | Single phosphonate ester |
Notes:
- Etidronic acid (HEDP): A well-studied bisphosphonate with a hydroxyl group on the central carbon, widely used in water treatment and medicine .
- Lauryl alcohol diphosphonic acid: Features a long alkyl chain, enhancing surfactant properties and metal ion chelation in organic media .
Physicochemical Properties
Table 2 compares critical physicochemical parameters:
Key Findings:
- Reactivity: The vinyl groups in "bis(1-methylethenyl)-diphosphonic acid" may confer higher reactivity in radical polymerization or crosslinking compared to HEDP or lauryl derivatives.
- Chelation Strength: HEDP’s hydroxyl group enhances its affinity for calcium ions, making it effective in preventing scale formation in water systems . In contrast, the vinyl groups in the target compound might prioritize transition metal chelation.
Biological Activity
Diphosphonic acid, bis(1-methylethenyl)-, also known as P,P'-bis(1-methylethenyl) diphosphonic acid, is a compound of significant interest due to its potential biological activities. This article explores its synthesis, biological properties, and relevant research findings, providing a comprehensive overview of its implications in medicinal chemistry.
Synthesis and Structural Characteristics
The synthesis of diphosphonic acids typically involves methodologies such as the Mannich-type reaction. This approach allows for the formation of phosphonate groups which are crucial for the biological activity of these compounds. The structural formula of diphosphonic acid, bis(1-methylethenyl)- is represented as follows:
The compound contains two phosphonate groups that contribute to its unique chemical properties and biological interactions.
Cytotoxicity Studies
Recent studies have evaluated the cytotoxic effects of various phosphonate derivatives, including diphosphonic acid, against different cancer cell lines. One notable study assessed the cytotoxicity of water-soluble phosphonates derived from natural compounds against human osteosarcoma cell lines (MG-63) and osteoblast precursor cells (MC3T3-E1) at concentrations ranging from 10 to 50 µM. The results indicated that many phosphonate derivatives exhibited low cytotoxicity towards MC3T3-E1 cells, suggesting a favorable safety profile for potential therapeutic applications .
Compound Number | Structural Formula | Aqueous Solubility (mg/mL) | Cytotoxicity (MG-63) |
---|---|---|---|
7a | - | 11 | Low |
7b | - | 8 | Low |
7c | - | 26 | Moderate |
8a | - | 3 | Low |
8b | - | 7 | Low |
8c | - | 7 | Low |
12 | - | 3 | Low |
The study highlighted that while some compounds showed promising solubility and low toxicity, the need for further investigation into their mechanisms of action remains critical .
The biological mechanisms through which diphosphonic acids exert their effects often involve interactions with cellular signaling pathways and enzymes. For instance, phosphonates can inhibit enzymes such as acetylcholinesterase (AChE), which plays a crucial role in neurotransmission. Inhibition of AChE can lead to increased levels of acetylcholine in synaptic clefts, affecting neuronal signaling and potentially leading to neurotoxicity if not regulated .
Case Studies and Research Findings
Several case studies have documented the effects of diphosphonic acids in various biological contexts:
- Neuroprotective Effects : Research has shown that certain phosphonates can protect neuronal cells from oxidative stress and apoptosis. This property could be beneficial in developing treatments for neurodegenerative diseases.
- Anticancer Properties : In vitro studies have demonstrated that diphosphonic acids may induce apoptosis in cancer cells through the activation of specific pathways. For example, compounds derived from natural products have been shown to enhance the efficacy of traditional chemotherapeutics .
- Antimicrobial Activity : Some phosphonates exhibit antimicrobial properties, making them candidates for developing new antibiotics or adjunct therapies to combat resistant bacterial strains .
Q & A
Q. Basic: What are the established synthetic routes for bis(1-methylethenyl) diphosphonic acid, and what are their comparative yields and purity profiles?
Answer:
Two primary synthetic routes are documented:
-
Route 1: Reaction of phosphorus trichloride (PCl₃) with glacial acetic acid (CH₃COOH) under controlled hydrolysis conditions. This method yields hydroxyethylidene diphosphonic acid (HEDP) via the reaction:
2PCl3+CH3COOH+5H2O→HEDP+6HCl. -
Route 2: Use of phosphorous acid (H₃PO₃) and acetic anhydride ((CH₃CO)₂O) with nitrous acid (HNO₂) as a catalyst, followed by hydrolysis.
Comparative studies indicate that Route 1 achieves higher yields (≈70–80%) but requires stringent handling of corrosive HCl byproducts. Route 2 offers better purity due to reduced side reactions but has lower overall yields (≈50–60%). Optimization of reaction temperature (15–25°C) and stoichiometric ratios is critical for both methods .
Q. Advanced: How do structural modifications in bis(1-methylethenyl) diphosphonic acid derivatives influence their acidity constants and chelation efficacy?
Answer:
Substituents significantly alter acidity constants (pKa) and metal-binding properties. For example:
- Electronegative groups (e.g., hydroxyl or imidazole in zoledronic acid) increase acidity by stabilizing deprotonated anions, lowering pKa values .
- Alkyl chain length in derivatives like dihexyl methylphosphonate reduces solubility but enhances lipophilicity, affecting membrane permeability in biological systems .
Studies on polymethylene diphosphonic acids show that increasing methylene spacers between phosphonate groups reduces acidity (e.g., pKa for methylene diphosphonic acid: \sim2.3 vs. tetramethylene: \sim3.8). This directly impacts chelation strength, with shorter spacers exhibiting stronger binding to divalent cations (e.g., Ca²⁺, Mg²⁺) .
Q. Basic: What analytical techniques are recommended for characterizing the purity and structural integrity of bis(1-methylethenyl) diphosphonic acid?
Answer:
- Nuclear Magnetic Resonance (NMR): ¹H and ³¹P NMR verify structural motifs (e.g., phosphonate peaks at δ 10–25 ppm in ³¹P NMR) .
- Mass Spectrometry (MS): High-resolution MS confirms molecular weight (e.g., m/z 206.03 for HEDP) .
- Elemental Analysis: Validates C, H, O, and P content (±0.3% deviation).
- Ion Chromatography: Quantifies residual chloride ions from synthesis .
Q. Advanced: What methodologies are employed to resolve discrepancies in reported biological activity data across different experimental models?
Answer:
Discrepancies in biological activity (e.g., analgesic effects in rats vs. bone resorption inhibition ) arise from:
- Dosage Variations: Dose-dependent effects (e.g., 5 mg/kg HEDP shows thermal analgesia, while 50 mg/kg affects mechanical pain thresholds) .
- Model-Specific Factors: Differences in species metabolism (e.g., rodent vs. human pharmacokinetics).
- Analytical Harmonization: Standardizing assays (e.g., tail-flick test vs. in vitro osteoclast assays) and controlling ionic strength/pH in studies to minimize confounding variables .
Q. Basic: What are the key thermodynamic parameters affecting the stability of bis(1-methylethenyl) diphosphonic acid in aqueous solutions?
Answer:
- Temperature: Elevated temperatures (25–50°C) marginally increase pKa values for weaker hydrogen dissociation (ΔH° ≈ -3.9 kcal/mol) .
- pH: Stability is optimal in mildly acidic conditions (pH 3–6), avoiding alkaline hydrolysis of phosphonate groups .
- Ionic Strength: High ionic strength (e.g., 0.1 M NaCl) lowers apparent pKa due to ion-pairing effects, altering metal-chelation dynamics .
Q. Advanced: How does the coordination chemistry of bis(1-methylethenyl) diphosphonic acid with metal ions influence its application in material science and agriculture?
Answer:
- Material Science: In photoresist developers, phosphonate-metal complexes (e.g., with Al³⁺ or Ti⁴⁺) modify surface adhesion and etching properties. Methylenediphosphonic acid enhances lithographic resolution by controlling metal ion diffusion .
- Agriculture: HEDP acts as a phosphorus fertilizer stabilizer, reducing soil fixation by forming soluble complexes with Fe³⁺ and Al³⁺. At 3% w/w, it decreases phosphorus fixation by 15% in calcareous soils .
Q. Basic: What safety protocols are recommended for handling bis(1-methylethenyl) diphosphonic acid in laboratory settings?
Answer:
- PPE: Acid-resistant gloves, goggles, and lab coats to prevent dermal/ocular exposure (risk code 36/37/38) .
- Ventilation: Use fume hoods during synthesis to mitigate HCl fumes (Route 1) .
- Storage: Airtight containers in dry, cool conditions to prevent hydrolysis .
Q. Advanced: What computational modeling approaches predict the reactivity and interaction mechanisms of bis(1-methylethenyl) diphosphonic acid in complex systems?
Answer:
- DFT Calculations: Model phosphonate group protonation states and metal-binding energies (e.g., Ca²⁺ binding ΔG ≈ -25 kcal/mol) .
- Molecular Dynamics (MD): Simulate aqueous solubility and membrane permeability of alkyl-substituted derivatives .
- QSAR Models: Correlate substituent electronegativity with pKa trends to design derivatives with tailored chelation strengths .
Properties
CAS No. |
177570-73-9 |
---|---|
Molecular Formula |
C6H12O5P2 |
Molecular Weight |
226.10 g/mol |
IUPAC Name |
[hydroxy(prop-1-en-2-yl)phosphoryl]oxy-prop-1-en-2-ylphosphinic acid |
InChI |
InChI=1S/C6H12O5P2/c1-5(2)12(7,8)11-13(9,10)6(3)4/h1,3H2,2,4H3,(H,7,8)(H,9,10) |
InChI Key |
SXOAILMFXPEWPE-UHFFFAOYSA-N |
Canonical SMILES |
CC(=C)P(=O)(O)OP(=O)(C(=C)C)O |
Related CAS |
177570-74-0 |
Origin of Product |
United States |
Disclaimer and Information on In-Vitro Research Products
Please be aware that all articles and product information presented on BenchChem are intended solely for informational purposes. The products available for purchase on BenchChem are specifically designed for in-vitro studies, which are conducted outside of living organisms. In-vitro studies, derived from the Latin term "in glass," involve experiments performed in controlled laboratory settings using cells or tissues. It is important to note that these products are not categorized as medicines or drugs, and they have not received approval from the FDA for the prevention, treatment, or cure of any medical condition, ailment, or disease. We must emphasize that any form of bodily introduction of these products into humans or animals is strictly prohibited by law. It is essential to adhere to these guidelines to ensure compliance with legal and ethical standards in research and experimentation.