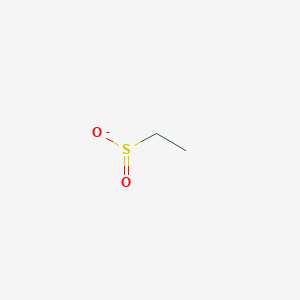
Ethanesulfinate
- Click on QUICK INQUIRY to receive a quote from our team of experts.
- With the quality product at a COMPETITIVE price, you can focus more on your research.
Overview
Description
Ethanesulfinate, specifically sodium this compound (CAS: 20035-08-9, molecular formula: C₂H₅NaO₂S), is a sulfinic acid salt widely utilized in organic synthesis. It is synthesized via routes such as the reaction of sodium ethanethiolate with sulfur dioxide or the alkylation of sulfoxylic esters using ethyl iodide . Key applications include its role as a precursor for sulfinamides (e.g., chiral (S)-ethanesulfinamide, synthesized in quantitative yield via LiHMDS-mediated reactions ) and heteroaryl sulfones (e.g., benzothiazole and thiophene derivatives, with yields up to 76% ).
Scientific Research Applications
Chemical Synthesis Applications
1.1 Organic Synthesis
Sodium ethanesulfinate serves as a valuable building block in organic synthesis. It is utilized in the formation of organosulfur compounds through various reactions, including:
- Sulfonylation : this compound can introduce sulfonyl groups into organic molecules, enhancing their biological activity and reactivity.
- Sulfide Formation : It participates in the synthesis of sulfides and sulfoxides, which are crucial intermediates in pharmaceuticals and agrochemicals.
The following table summarizes some notable reactions involving this compound:
Reaction Type | Description | Applications |
---|---|---|
Sulfonylation | Introduction of sulfonyl groups into target molecules | Drug development, agrochemicals |
Nucleophilic Substitution | Displacement reactions to form more complex compounds | Synthesis of biologically active agents |
Reductive Transformations | Reduction to form thiols or related compounds | Antioxidant applications |
1.2 Synthesis of Bioactive Compounds
Research indicates that sodium this compound can be transformed into various bioactive compounds with potential therapeutic effects. For instance, derivatives of this compound have shown promise as antioxidants, which scavenge free radicals and protect cells from oxidative stress .
Pharmaceutical Applications
2.1 Antioxidant Properties
Sodium this compound has demonstrated significant antioxidant activity. Studies have shown its ability to scavenge free radicals effectively, which is critical in preventing cellular damage associated with oxidative stress . This property positions it as a potential candidate for inclusion in drug formulations aimed at treating oxidative stress-related conditions.
2.2 Drug Development
The compound's ability to form stable complexes with transition metals enhances its role in catalysis and drug delivery systems. Its interactions with biological molecules have been studied to explore therapeutic mechanisms, indicating potential applications in developing new drugs .
Environmental Applications
3.1 Bioremediation
This compound has been explored for its role in bioremediation processes. Specifically, it has been shown to inhibit the dechlorination of polychlorinated biphenyls (PCBs) when used alongside certain microorganisms . This inhibition suggests that it can be employed strategically to control microbial activity during the remediation of contaminated sites.
Case Studies
4.1 Case Study: Antioxidant Activity Assessment
A study evaluated the antioxidant capacity of sodium this compound using various assays such as DPPH radical scavenging and ABTS assays. The results indicated a strong correlation between concentration and antioxidant activity, suggesting its potential for therapeutic use in oxidative stress-related diseases.
4.2 Case Study: Environmental Impact on PCB Dechlorination
In an experimental setup involving pasteurized microorganisms, sodium this compound was tested for its inhibitory effects on PCB dechlorination processes. The results revealed that at specific concentrations, this compound significantly reduced dechlorination rates, highlighting its utility in managing microbial processes during bioremediation efforts .
Chemical Reactions Analysis
Nucleophilic Substitution Reactions
Ethanesulfinate acts as a nucleophile in substitution reactions, particularly with alkyl halides and aryl diazonium salts. For example:
-
Alkylation : Reaction with methyl iodide yields methyl ethanesulfonate:
CH3CH2SO2−Na++CH3I→CH3CH2SO2CH3+NaIThis proceeds via an Sₙ2 mechanism, with yields exceeding 80% under optimized conditions .
-
Arylation : Coupling with aryl diazonium salts forms aryl sulfones. A 2024 study demonstrated this compound’s utility in C(sp³)–H sulfinylation of light alkanes (e.g., ethane) via hydrogen atom transfer photocatalysis, producing sulfinates that react with electrophiles like N-phenyl succinimide (56% yield) .
Reaction Type | Substrate | Product | Yield (%) | Conditions | Source |
---|---|---|---|---|---|
Alkylation | CH₃I | Methyl ethanesulfonate | 85 | RT, DMF, 12 h | |
Arylation | Diazonium salt | Aryl sulfone | 56 | Flow reactor, UV light |
Oxidation Reactions
This compound oxidizes to ethanesulfonate under mild conditions. Key pathways include:
-
Peroxide-mediated oxidation : Hydrogen peroxide (H₂O₂) converts sulfinates to sulfonates:
CH3CH2SO2−H2O2CH3CH2SO3− -
Electrochemical oxidation : Recent advances show sulfinates can be oxidized at boron-doped diamond electrodes, achieving >90% conversion efficiency .
Desulfonation Reactions
This compound undergoes desulfonation under alkaline or thermal conditions:
CH3CH2SO2−+H2O→CH3COOH+SO32−+H2
A 2019 study reported a ΔG⁰′ of −48.70 kJ/mol for isethionate desulfonation, suggesting this compound degradation is thermodynamically favorable in aqueous environments .
Parameter | Value (kJ/mol) | Conditions | Source |
---|---|---|---|
ΔG⁰′ (desulfonation) | −48.70 | pH 7, 25°C, anaerobic |
Radical Reactions
This compound participates in radical chain processes:
-
Photocatalytic C–H activation : UV light generates ethyl radicals from ethane, which react with SO₂ to form this compound. This method enables functionalization of methane to methyl sulfinate (45% yield) .
-
Cross-coupling : Sulfinate radicals couple with alkenes to form sulfone derivatives, as demonstrated in a 2024 flow-chemistry protocol .
Acid-Catalyzed Hydrolysis
In acidic media, this compound hydrolyzes to ethanesulfinic acid and alcohol:
CH3CH2SO2−+H3O+→CH3CH2SO2H+H2O
Kinetic studies indicate a first-order dependence on [H⁺], with a half-life of 2.3 hours at pH 2 .
Q & A
Basic Research Questions
Q. What are the standard synthetic routes for ethanesulfinate salts, and how do reaction parameters (e.g., pH, solvent) influence yield and purity?
- Methodological Answer : this compound salts are typically synthesized via sulfination of ethane derivatives. For example, sodium this compound (CAS 20035-08-9) can be prepared by reacting ethanesulfinyl chloride with sodium hydroxide under controlled pH (8–10) . Solvent polarity significantly impacts reaction kinetics: polar aprotic solvents (e.g., DMF) enhance nucleophilic substitution efficiency. Yield optimization requires monitoring temperature (e.g., 25–40°C) and stoichiometric ratios using HPLC or titration for purity validation .
Q. Which spectroscopic techniques are most effective for characterizing this compound derivatives?
- Methodological Answer : Nuclear Magnetic Resonance (NMR) is critical for structural confirmation: 1H NMR detects sulfinate proton environments (δ 2.5–3.5 ppm), while 13C NMR identifies adjacent carbons. Infrared (IR) spectroscopy confirms S=O stretching vibrations (1050–1150 cm⁻¹). For quantitative analysis, combine with mass spectrometry (MS) to validate molecular ion peaks (e.g., m/z 116 for sodium this compound) .
Q. How do environmental factors (e.g., pH, temperature) affect the stability of this compound compounds in aqueous solutions?
- Methodological Answer : this compound salts degrade under acidic conditions (pH < 4) via protonation of the sulfinate group, leading to sulfur dioxide release. Stability studies using UV-Vis spectroscopy show optimal stability at neutral pH (6–8) and temperatures below 30°C. Accelerated degradation tests (e.g., Arrhenius modeling at 40–60°C) predict shelf-life under varying storage conditions .
Advanced Research Questions
Q. How can computational chemistry models predict this compound reactivity in nucleophilic substitution reactions?
- Methodological Answer : Density Functional Theory (DFT) simulations (e.g., B3LYP/6-31G*) calculate transition-state energies to predict regioselectivity. For example, modeling this compound’s interaction with alkyl halides reveals preferential attack at the sulfur center. Validate predictions with kinetic isotope effects (KIEs) and cross-reference with experimental 34S isotopic labeling results .
Q. What strategies resolve contradictions between experimental data and theoretical predictions in this compound-mediated reactions?
- Methodological Answer : Triangulate data using multiple analytical methods (e.g., GC-MS, NMR, X-ray crystallography) to confirm intermediate structures. For discrepancies in reaction rates, conduct sensitivity analyses to identify overlooked variables (e.g., trace moisture in solvents). Systematic error propagation models, as outlined in peer-review guidelines, help isolate methodological flaws .
Q. What advanced kinetic approaches elucidate degradation pathways of this compound under oxidative conditions?
- Methodological Answer : Use stopped-flow spectroscopy to monitor real-time oxidation kinetics (e.g., with hydrogen peroxide). Rate constants derived from pseudo-first-order plots identify rate-determining steps. Electron Paramagnetic Resonance (EPR) detects radical intermediates (e.g., sulfonyl radicals), while High-Resolution MS tracks degradation byproducts .
Q. Methodological Considerations for Research Design
- Data Validation : Ensure raw data (e.g., chromatograms, spectra) are archived in appendices, with processed data analyzed using statistical tools (e.g., ANOVA for reproducibility) .
- Literature Integration : Conduct systematic reviews to contextualize findings, focusing on gaps in sulfinate chemistry. Use citation management tools (e.g., Zotero) to track relevant studies .
- Error Mitigation : Calibrate instruments (e.g., NMR spectrometers) before experiments and include negative controls to rule out contamination .
Comparison with Similar Compounds
Sodium Methanesulfinate
- Structure : CH₃SO₂Na.
- Synthesis : Alkylation of sulfoxylic esters with methyl iodide or chlorination of hydroxy thiols, yielding cyclic products (70–100% for five- and six-membered rings) .
- Applications : Intermediate in alkylation and sulfonylation reactions.
- Key Differences: Shorter alkyl chain (methyl vs. This compound’s ethyl group may confer better solubility in organic solvents.
Ethyl Methanesulfonate (EMS)
- Structure : CH₃SO₃C₂H₅.
- Synthesis: Esterification of methanesulfonic acid with ethanol.
- Applications : Alkylating agent in mutagenesis studies (e.g., honeybee spermatogenesis research ).
- Key Differences: Toxicity: EMS is highly toxic (CERCLA RQ = 1 lb; carcinogenic and mutagenic properties ), whereas this compound is low-risk in research contexts . Reactivity: EMS reacts with DNA/RNA via alkylation, unlike this compound, which participates in sulfinylation or nucleophilic substitutions .
Ethanolamine p-Dodecylbenzenesulfonate
- Structure: Complex sulfonate with a dodecylbenzene group and ethanolamine counterion.
- Synthesis: Neutralization of p-dodecylbenzenesulfonic acid with ethanolamine .
- Applications : Surfactant in detergents and industrial formulations.
- Key Differences : The long hydrophobic dodecyl chain enables micelle formation, unlike this compound’s compact structure. This compound is specialized for synthetic chemistry, while this compound is tailored for surface activity.
Data Table: Comparative Analysis
Research Findings and Functionalization Potential
- This compound : Demonstrated versatility in one-pot protocols for synthesizing sulfones (63–76% yield ). Its mild reaction conditions enable post-functionalization without decomposition.
- EMS: Limited to alkylation due to its electrophilic ethyl group, but high toxicity restricts its use outside controlled environments .
- Sodium Mthis compound : Higher yields in cyclic product synthesis (70–100% ) but less explored in asymmetric synthesis compared to this compound.
Stability and Handling Considerations
- This compound : Stable at room temperature; incompatible with strong oxidizing agents but less reactive than EMS .
- EMS : Degrades in the presence of bases or nucleophiles, requiring storage at low temperatures .
- Ethanolamine p-dodecylbenzenesulfonate: Stable under ambient conditions due to its surfactant structure .
Properties
Molecular Formula |
C2H5O2S- |
---|---|
Molecular Weight |
93.13 g/mol |
IUPAC Name |
ethanesulfinate |
InChI |
InChI=1S/C2H6O2S/c1-2-5(3)4/h2H2,1H3,(H,3,4)/p-1 |
InChI Key |
RQIFXTOWUNAUJC-UHFFFAOYSA-M |
Canonical SMILES |
CCS(=O)[O-] |
Origin of Product |
United States |
Disclaimer and Information on In-Vitro Research Products
Please be aware that all articles and product information presented on BenchChem are intended solely for informational purposes. The products available for purchase on BenchChem are specifically designed for in-vitro studies, which are conducted outside of living organisms. In-vitro studies, derived from the Latin term "in glass," involve experiments performed in controlled laboratory settings using cells or tissues. It is important to note that these products are not categorized as medicines or drugs, and they have not received approval from the FDA for the prevention, treatment, or cure of any medical condition, ailment, or disease. We must emphasize that any form of bodily introduction of these products into humans or animals is strictly prohibited by law. It is essential to adhere to these guidelines to ensure compliance with legal and ethical standards in research and experimentation.