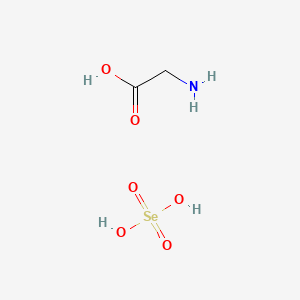
Triglycine selenate
- Click on QUICK INQUIRY to receive a quote from our team of experts.
- With the quality product at a COMPETITIVE price, you can focus more on your research.
Overview
Description
Triglycine selenate, chemically represented as ((\text{NH}_2\text{CH}_2\text{COOH})_3\text{H}_2\text{SeO}_4), is a hydrogen-bonded ferroelectric compound. It undergoes a structural phase transition at approximately 295K, transitioning from a paraelectric to a ferroelectric phase. This compound is closely related to triglycine sulfate and is known for its unique ferroelectric properties, which make it valuable in various scientific and industrial applications .
Preparation Methods
Synthetic Routes and Reaction Conditions: Triglycine selenate can be synthesized by slow evaporation of a saturated aqueous solution containing glycine and selenic acid in stoichiometric proportions. The process involves dissolving glycine and selenic acid in water, followed by slow evaporation at room temperature to form single crystals .
Industrial Production Methods: Industrial production of this compound follows similar principles but on a larger scale. The controlled evaporation process ensures the formation of high-quality crystals. The purity of the starting materials and the control of environmental conditions are crucial for obtaining consistent and high-quality this compound crystals .
Chemical Reactions Analysis
Types of Reactions: Triglycine selenate primarily undergoes phase transitions rather than traditional chemical reactions like oxidation or reduction. it can participate in hydrogen bonding and proton transfer reactions due to its glycine components .
Common Reagents and Conditions: The primary reagents involved in the synthesis and reactions of this compound are glycine and selenic acid. The reaction conditions typically involve aqueous solutions and controlled temperature environments .
Major Products: The major product of the synthesis is this compound crystals. No significant by-products are formed under controlled conditions .
Scientific Research Applications
Triglycine selenate has a wide range of applications in scientific research:
Ferroelectric Studies: Due to its ferroelectric properties, this compound is extensively studied for its phase transition behavior and dielectric properties
Material Science: It serves as a model compound for studying hydrogen-bonded ferroelectrics and their applications in sensors and actuators.
Biological Research: The compound’s unique properties make it useful in studying protein crystallization and other biological processes involving hydrogen bonding.
Medical Applications: Research is ongoing to explore its potential in medical diagnostics and therapeutic applications due to its unique dielectric properties.
Mechanism of Action
The ferroelectric behavior of triglycine selenate is primarily due to the hydrogen bonding and proton transfer between the glycine molecules and the selenate group. The phase transition from paraelectric to ferroelectric involves a change in the symmetry of the crystal structure, which affects its dielectric properties. The molecular targets and pathways involved include the glycine molecules and the hydrogen bonds that stabilize the crystal structure .
Comparison with Similar Compounds
Triglycine Sulfate: Chemically similar to triglycine selenate but contains sulfate instead of selenate. .
Triglycine Fluoroberyllate: Another member of the triglycine family, known for its unique ferroelectric properties.
Uniqueness: this compound is unique due to the presence of the selenate group, which significantly influences its ferroelectric properties. The specific heat peak of this compound is six times larger than that of triglycine sulfate, making it particularly interesting for thermal studies .
Properties
CAS No. |
920-25-2 |
---|---|
Molecular Formula |
C2H7NO6Se |
Molecular Weight |
220.05 g/mol |
IUPAC Name |
2-aminoacetic acid;selenic acid |
InChI |
InChI=1S/C2H5NO2.H2O4Se/c3-1-2(4)5;1-5(2,3)4/h1,3H2,(H,4,5);(H2,1,2,3,4) |
InChI Key |
MFPXBAPCIMYWHZ-UHFFFAOYSA-N |
Canonical SMILES |
C(C(=O)O)N.O[Se](=O)(=O)O |
Origin of Product |
United States |
Disclaimer and Information on In-Vitro Research Products
Please be aware that all articles and product information presented on BenchChem are intended solely for informational purposes. The products available for purchase on BenchChem are specifically designed for in-vitro studies, which are conducted outside of living organisms. In-vitro studies, derived from the Latin term "in glass," involve experiments performed in controlled laboratory settings using cells or tissues. It is important to note that these products are not categorized as medicines or drugs, and they have not received approval from the FDA for the prevention, treatment, or cure of any medical condition, ailment, or disease. We must emphasize that any form of bodily introduction of these products into humans or animals is strictly prohibited by law. It is essential to adhere to these guidelines to ensure compliance with legal and ethical standards in research and experimentation.