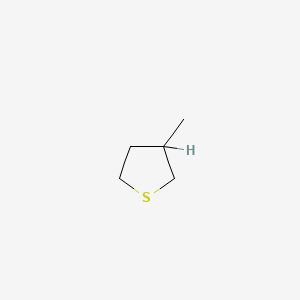
3-Methylthiolane
Overview
Description
3-Methylthiolane is a useful research compound. Its molecular formula is C5H10S and its molecular weight is 102.2 g/mol. The purity is usually 95%.
BenchChem offers high-quality this compound suitable for many research applications. Different packaging options are available to accommodate customers' requirements. Please inquire for more information about this compound including the price, delivery time, and more detailed information at info@benchchem.com.
Scientific Research Applications
Synthesis Applications
3-Methylthiolane and its derivatives have been utilized in various synthesis processes. For example, 2-(3-nitro-4-methoxyformyl)-aryl-1,3-dithiolanes were synthesized using 3-nitro-4-methyl benzoic acid, demonstrating the role of this compound derivatives in spice synthesis. These compounds were found to have sweet spice aromas, showcasing the potential in flavor and fragrance industries (Wang Ting-ting, 2010).
Medicinal Chemistry
In medicinal chemistry, α-lipoic acid-based derivatives, including those with 1,2-dithiolane structures, have been developed as PPARγ agonists. These derivatives exhibit potential for treating inflammatory skin diseases, highlighting the therapeutic relevance of this compound related compounds (M. Venkatraman et al., 2004).
Electrochemistry
The potential applications of poly-3-methylthiophene (P3MT) in electrochemistry, particularly in battery and fuel cell research, have been explored. This research underscores the utility of this compound in developing advanced materials for energy applications (H. B. Mark et al., 2000).
Corrosion Inhibition
Ketene dithioacetal derivatives, including this compound compounds, have demonstrated effectiveness in inhibiting copper corrosion in nitric acid solutions. This points to their potential use in industrial applications to protect metals from corrosion (A. Fiala et al., 2007).
Ecological and Environmental Applications
Synthetic predator odors, including compounds like 3,3-dimethyl-1,2-dithiolane (DMDIT), have been tested for their effectiveness in eliciting behavioral responses in pests such as roof rats. This research indicates potential applications of this compound in pest control and environmental management (Michael D. Burwash et al., 1998).
Allelopathy
Compounds like 4-methylthio-1,2-dithiolane, derived from aquatic macrophytes, have been identified as allelopathic compounds. These substances can inhibit the growth of epiphytic phytoplankton, suggesting a role in ecological balance and potential use in managing aquatic ecosystems (S. Wium-Andersen et al., 1982).
Material Science
Dithiolane-containing polymers have been used to construct responsive and dynamic networks in material science. This research highlights the adaptability and self-healing properties of such materials, which could be relevant in various technological applications (Xiangyi Zhang & R. Waymouth, 2017).
Safety and Hazards
Properties
IUPAC Name |
3-methylthiolane | |
---|---|---|
Source | PubChem | |
URL | https://pubchem.ncbi.nlm.nih.gov | |
Description | Data deposited in or computed by PubChem | |
InChI |
InChI=1S/C5H10S/c1-5-2-3-6-4-5/h5H,2-4H2,1H3 | |
Source | PubChem | |
URL | https://pubchem.ncbi.nlm.nih.gov | |
Description | Data deposited in or computed by PubChem | |
InChI Key |
DLABLFPCTXRQMY-UHFFFAOYSA-N | |
Source | PubChem | |
URL | https://pubchem.ncbi.nlm.nih.gov | |
Description | Data deposited in or computed by PubChem | |
Canonical SMILES |
CC1CCSC1 | |
Source | PubChem | |
URL | https://pubchem.ncbi.nlm.nih.gov | |
Description | Data deposited in or computed by PubChem | |
Molecular Formula |
C5H10S | |
Source | PubChem | |
URL | https://pubchem.ncbi.nlm.nih.gov | |
Description | Data deposited in or computed by PubChem | |
DSSTOX Substance ID |
DTXSID20871097 | |
Record name | 3-Methylthiolane | |
Source | EPA DSSTox | |
URL | https://comptox.epa.gov/dashboard/DTXSID20871097 | |
Description | DSSTox provides a high quality public chemistry resource for supporting improved predictive toxicology. | |
Molecular Weight |
102.20 g/mol | |
Source | PubChem | |
URL | https://pubchem.ncbi.nlm.nih.gov | |
Description | Data deposited in or computed by PubChem | |
CAS No. |
4740-00-5 | |
Record name | Thiophene, tetrahydro-3-methyl- | |
Source | ChemIDplus | |
URL | https://pubchem.ncbi.nlm.nih.gov/substance/?source=chemidplus&sourceid=0004740005 | |
Description | ChemIDplus is a free, web search system that provides access to the structure and nomenclature authority files used for the identification of chemical substances cited in National Library of Medicine (NLM) databases, including the TOXNET system. | |
Record name | 3-Methylthiolane | |
Source | EPA DSSTox | |
URL | https://comptox.epa.gov/dashboard/DTXSID20871097 | |
Description | DSSTox provides a high quality public chemistry resource for supporting improved predictive toxicology. | |
Record name | 3-methylthiolane | |
Source | European Chemicals Agency (ECHA) | |
URL | https://echa.europa.eu/information-on-chemicals | |
Description | The European Chemicals Agency (ECHA) is an agency of the European Union which is the driving force among regulatory authorities in implementing the EU's groundbreaking chemicals legislation for the benefit of human health and the environment as well as for innovation and competitiveness. | |
Explanation | Use of the information, documents and data from the ECHA website is subject to the terms and conditions of this Legal Notice, and subject to other binding limitations provided for under applicable law, the information, documents and data made available on the ECHA website may be reproduced, distributed and/or used, totally or in part, for non-commercial purposes provided that ECHA is acknowledged as the source: "Source: European Chemicals Agency, http://echa.europa.eu/". Such acknowledgement must be included in each copy of the material. ECHA permits and encourages organisations and individuals to create links to the ECHA website under the following cumulative conditions: Links can only be made to webpages that provide a link to the Legal Notice page. | |
Retrosynthesis Analysis
AI-Powered Synthesis Planning: Our tool employs the Template_relevance Pistachio, Template_relevance Bkms_metabolic, Template_relevance Pistachio_ringbreaker, Template_relevance Reaxys, Template_relevance Reaxys_biocatalysis model, leveraging a vast database of chemical reactions to predict feasible synthetic routes.
One-Step Synthesis Focus: Specifically designed for one-step synthesis, it provides concise and direct routes for your target compounds, streamlining the synthesis process.
Accurate Predictions: Utilizing the extensive PISTACHIO, BKMS_METABOLIC, PISTACHIO_RINGBREAKER, REAXYS, REAXYS_BIOCATALYSIS database, our tool offers high-accuracy predictions, reflecting the latest in chemical research and data.
Strategy Settings
Precursor scoring | Relevance Heuristic |
---|---|
Min. plausibility | 0.01 |
Model | Template_relevance |
Template Set | Pistachio/Bkms_metabolic/Pistachio_ringbreaker/Reaxys/Reaxys_biocatalysis |
Top-N result to add to graph | 6 |
Feasible Synthetic Routes
Disclaimer and Information on In-Vitro Research Products
Please be aware that all articles and product information presented on BenchChem are intended solely for informational purposes. The products available for purchase on BenchChem are specifically designed for in-vitro studies, which are conducted outside of living organisms. In-vitro studies, derived from the Latin term "in glass," involve experiments performed in controlled laboratory settings using cells or tissues. It is important to note that these products are not categorized as medicines or drugs, and they have not received approval from the FDA for the prevention, treatment, or cure of any medical condition, ailment, or disease. We must emphasize that any form of bodily introduction of these products into humans or animals is strictly prohibited by law. It is essential to adhere to these guidelines to ensure compliance with legal and ethical standards in research and experimentation.