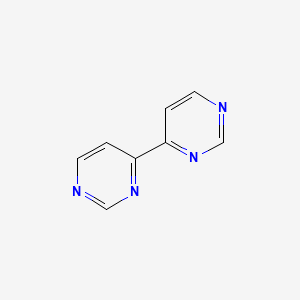
4,4'-Bipyrimidine
Overview
Description
4,4’-Bipyrimidine is an organic compound with the molecular formula C8H6N4 . It is a popular scaffold primarily employed as a linker in 3D self-assembled architectures such as metallo-organic frameworks or as a connector in 2D networks .
Synthesis Analysis
Several synthetic strategies have been described to access 4,4’-bipyridines functionalized at various positions . These easily scalable reactions have been used to introduce a range of alkyl substituents at positions 2 and 2’ or 3 and 3’ and at positions 2,2’ and 6,6’ in the case of tetra-functionalization .
Molecular Structure Analysis
The molecular weight of 4,4’-Bipyrimidine is 158.16 g/mol . The InChIKey of the compound is TZLCPYJWWDXRMH-UHFFFAOYSA-N . The compound has a topological polar surface area of 51.6 Ų .
Chemical Reactions Analysis
The photochemistry of 4,4’-bipyridine has been studied, including the nanosecond absorption and Raman study of the hydrogen atom abstraction from methanol and 2-propanol .
Physical And Chemical Properties Analysis
4,4’-Bipyrimidine has a molecular weight of 158.16 g/mol . It has a hydrogen bond donor count of 0 and a hydrogen bond acceptor count of 4 . The compound has a rotatable bond count of 1 .
Scientific Research Applications
Building Blocks for Medium- and Environment-Responsive Compounds and Materials
Mono- and di-quaternized 4,4′-bipyridine derivatives are a family of heterocyclic compounds that have been employed in numerous applications. These applications correspond to various disciplines of research and technology. Two key features of these 4,4′-bipyridine-based derivatives are exploited: their redox activity and their electrochromic aptitude .
Molecular Motors, Machines, and Switches
In recent years, the derivatives of 4,4′-bipyridine have been employed in numerous high-tech applications including molecular motors, machines, and switches .
Supramolecular Chemistry
4,4′-Bipyridine is highly abundant in supramolecular chemistry, where it mainly serves as an electro- and photo-sensitive building block .
Metal-Organic Frameworks (MOFs)
A new metal–organic framework (MOF), namely, { [Ni (BPS) (4,4′-bipy)] n }, (BPS = 4,4′-bibenzoic acid-2,2′-sulfone, bipy = 4,4-Bipyridine) ( 1 ), with 3D framework has been synthesized under solvothermal condition .
Magnetic Materials
The magnetic experiments reveal that 1 exhibits typical ferromagnetic exchange in Ni (II) ions .
Coformers and Ligands in Coordination Chemistry
Two bipyridine isomers (2,2′- and 4,4′-), used as coformers and ligands in coordination chemistry .
Mechanism of Action
- Its primary targets are not well-documented in the literature. However, it is commonly used as a ligand in coordination chemistry, where it coordinates with metal ions to form complexes. These complexes can have various applications, including catalysis, sensing, and material science .
Target of Action
Safety and Hazards
In case of inhalation, move the victim into fresh air. If breathing is difficult, give oxygen. If not breathing, give artificial respiration and consult a doctor immediately . Following skin contact, take off contaminated clothing immediately. Wash off with soap and plenty of water. Consult a doctor .
Future Directions
properties
IUPAC Name |
4-pyrimidin-4-ylpyrimidine | |
---|---|---|
Source | PubChem | |
URL | https://pubchem.ncbi.nlm.nih.gov | |
Description | Data deposited in or computed by PubChem | |
InChI |
InChI=1S/C8H6N4/c1-3-9-5-11-7(1)8-2-4-10-6-12-8/h1-6H | |
Source | PubChem | |
URL | https://pubchem.ncbi.nlm.nih.gov | |
Description | Data deposited in or computed by PubChem | |
InChI Key |
TZLCPYJWWDXRMH-UHFFFAOYSA-N | |
Source | PubChem | |
URL | https://pubchem.ncbi.nlm.nih.gov | |
Description | Data deposited in or computed by PubChem | |
Canonical SMILES |
C1=CN=CN=C1C2=NC=NC=C2 | |
Source | PubChem | |
URL | https://pubchem.ncbi.nlm.nih.gov | |
Description | Data deposited in or computed by PubChem | |
Molecular Formula |
C8H6N4 | |
Source | PubChem | |
URL | https://pubchem.ncbi.nlm.nih.gov | |
Description | Data deposited in or computed by PubChem | |
DSSTOX Substance ID |
DTXSID40178959 | |
Record name | 4,4'-Bipyrimidine | |
Source | EPA DSSTox | |
URL | https://comptox.epa.gov/dashboard/DTXSID40178959 | |
Description | DSSTox provides a high quality public chemistry resource for supporting improved predictive toxicology. | |
Molecular Weight |
158.16 g/mol | |
Source | PubChem | |
URL | https://pubchem.ncbi.nlm.nih.gov | |
Description | Data deposited in or computed by PubChem | |
Product Name |
4,4'-Bipyrimidine | |
CAS RN |
2426-94-0 | |
Record name | 4,4'-Bipyrimidine | |
Source | ChemIDplus | |
URL | https://pubchem.ncbi.nlm.nih.gov/substance/?source=chemidplus&sourceid=0002426940 | |
Description | ChemIDplus is a free, web search system that provides access to the structure and nomenclature authority files used for the identification of chemical substances cited in National Library of Medicine (NLM) databases, including the TOXNET system. | |
Record name | 4,4'-Bipyrimidine | |
Source | EPA DSSTox | |
URL | https://comptox.epa.gov/dashboard/DTXSID40178959 | |
Description | DSSTox provides a high quality public chemistry resource for supporting improved predictive toxicology. | |
Retrosynthesis Analysis
AI-Powered Synthesis Planning: Our tool employs the Template_relevance Pistachio, Template_relevance Bkms_metabolic, Template_relevance Pistachio_ringbreaker, Template_relevance Reaxys, Template_relevance Reaxys_biocatalysis model, leveraging a vast database of chemical reactions to predict feasible synthetic routes.
One-Step Synthesis Focus: Specifically designed for one-step synthesis, it provides concise and direct routes for your target compounds, streamlining the synthesis process.
Accurate Predictions: Utilizing the extensive PISTACHIO, BKMS_METABOLIC, PISTACHIO_RINGBREAKER, REAXYS, REAXYS_BIOCATALYSIS database, our tool offers high-accuracy predictions, reflecting the latest in chemical research and data.
Strategy Settings
Precursor scoring | Relevance Heuristic |
---|---|
Min. plausibility | 0.01 |
Model | Template_relevance |
Template Set | Pistachio/Bkms_metabolic/Pistachio_ringbreaker/Reaxys/Reaxys_biocatalysis |
Top-N result to add to graph | 6 |
Feasible Synthetic Routes
Q & A
A: 4,4′-Bipyrimidine has the molecular formula C8H6N4 and a molecular weight of 158.16 g/mol. []
ANone: Numerous studies utilize various spectroscopic techniques to characterize 4,4′-bipyrimidine and its derivatives. These techniques include:
- NMR Spectroscopy (1H, 13C, 195Pt): Used to determine structural features, analyze isomer ratios in metal complexes, and investigate electronic effects of substituents on the ligand and metal centers. [, , , , ]
- UV-Vis/NIR Spectroscopy: Employed to analyze electronic transitions, particularly metal-to-ligand charge transfer (MLCT) bands, in 4,4′-bipyrimidine metal complexes. This data provides insights into the energy levels and redox properties of these complexes. [, , , , , , ]
- Resonance Raman Spectroscopy: Utilized to study the vibrational modes and excited-state dynamics of ruthenium(II) complexes containing 4,4′-bipyrimidine ligands. []
- Electron Paramagnetic Resonance (EPR) Spectroscopy: Used to characterize radical cation intermediates formed during the reduction of 4,4′-bipyrimidine derivatives and their metal complexes. This technique provides information about the electron spin distribution and electronic structure of these species. [, ]
A: The stability of 4,4′-bipyrimidine metal complexes is influenced by the metal ion, its oxidation state, and the nature of the substituents on the bipyrimidine ring. For example, ruthenium(II) complexes with substituted 4,4′-bipyrimidine ligands exhibit high energy excited states, making them suitable for incorporation into polynuclear arrays. [] The presence of electron-withdrawing substituents on the bipyrimidine ring can stabilize the LUMO of the ligand and influence the stability of its reduced forms. []
ANone: 4,4′-Bipyrimidine metal complexes have shown promising activity in various catalytic reactions, including:
- Electrochemical CO2 Reduction: Manganese complexes with 4,4′-bipyrimidine ligands have been investigated for their ability to catalyze the electrochemical reduction of CO2 to CO. Computational studies suggest that these complexes have the potential for reduced overpotential and improved catalytic activity. []
- Formic Acid Dehydrogenation: Iridium complexes containing 4,4′-bipyrimidine ligands, specifically those with hydroxyl groups at the ortho positions, demonstrate high efficiency in catalyzing formic acid dehydrogenation for hydrogen generation. [, ]
- Epoxidation of Olefins: Molybdenum oxobisperoxo complexes with pyrazolylpyridine ligands, structurally related to 4,4′-bipyrimidine, have shown activity as catalysts for olefin epoxidation. []
ANone: Computational chemistry plays a vital role in studying 4,4′-bipyrimidine systems:
- Density Functional Theory (DFT): DFT calculations are employed to investigate reaction mechanisms, predict electrochemical properties, and elucidate the role of substituents and solvent effects in catalytic reactions involving 4,4′-bipyrimidine metal complexes. [, , ]
- CNDO/S Configuration Interaction Calculations: These calculations help rationalize the electronic spectra and assign transitions observed in UV-Vis/NIR studies of reduced forms of 4,4′-bipyrimidine and its isomers. []
- HMO/McLachlan Calculations: These calculations are employed to interpret EPR spectra and determine spin population distributions in radical cation intermediates of 4,4′-bipyrimidine derivatives. []
ANone: Structural modifications of 4,4′-bipyrimidine significantly impact its coordination behavior and the properties of the resulting metal complexes:
- Substituent Effects: Introducing electron-donating or -withdrawing groups at the 6,6′-positions of 4,4′-bipyrimidine can fine-tune the ligand's π-accepting ability, influencing the energy levels and electrochemical properties of metal complexes. [, , , ] For instance, 6,6′-disubstituted 4,4′-bipyrimidines are stronger π-acceptors than the unsubstituted parent ligand, leading to red-shifted MLCT absorptions in their metal complexes. []
- Steric Effects: Bulky substituents can influence the ligand's conformation and its ability to chelate metal ions, impacting the stability and structure of the resulting complexes. [, ]
- Bridging vs. Chelating Coordination: 4,4′-Bipyrimidine can act as a bridging or chelating ligand, depending on factors such as the metal ion, counterion, and reaction conditions. [, , , ]
ANone: A range of analytical techniques are utilized for characterizing 4,4′-bipyrimidine and its derivatives, including:
- X-ray Crystallography: This technique provides detailed information about the three-dimensional structure and packing arrangements of 4,4′-bipyrimidine metal complexes in the solid state. [, , , , , , , , , , , ]
- Cyclic Voltammetry: This electrochemical technique is used to investigate the redox properties of 4,4′-bipyrimidine and its metal complexes, providing information about their electron transfer behavior. [, , , ]
- Thermogravimetric Analysis (TGA): TGA is used to analyze the thermal stability and decomposition patterns of 4,4′-bipyrimidine metal complexes. []
A: While specific data on the environmental impact of 4,4′-bipyrimidine is limited, studies on bioremediation of polycyclic aromatic hydrocarbons (PAHs) identified 4,4′-bipyrimidine as a biointermediate. [] This finding suggests that 4,4′-bipyrimidine may be subject to microbial degradation in specific environmental contexts. Further research is needed to understand its persistence, bioaccumulation potential, and potential effects on ecosystems.
ANone: Research on 4,4′-bipyrimidine and its derivatives has progressed through several key milestones:
- Early Synthesis and Coordination Chemistry: The synthesis and coordination chemistry of 4,4′-bipyrimidine were first explored in the latter half of the 20th century, laying the groundwork for its use as a versatile ligand in coordination chemistry. [, ]
- Development of Substituted Derivatives: The synthesis and characterization of various substituted 4,4′-bipyrimidine derivatives allowed researchers to fine-tune the ligand's electronic and steric properties, leading to the development of complexes with tailored properties for specific applications. [, , , ]
- Exploration of Catalytic Applications: More recently, 4,4′-bipyrimidine metal complexes have gained attention for their potential in catalytic applications, including CO2 reduction, formic acid dehydrogenation, and potentially other reactions. [, , ]
ANone: The study of 4,4′-bipyrimidine and its derivatives exemplifies cross-disciplinary research, integrating:
- Coordination Chemistry: Understanding the coordination behavior of 4,4′-bipyrimidine with various metal ions under different conditions is crucial for designing complexes with specific properties. [, , , , ]
- Catalysis: The exploration of 4,4′-bipyrimidine metal complexes as catalysts for reactions like CO2 reduction and formic acid dehydrogenation highlights the intersection of this field with sustainable energy research. [, , ]
- Materials Science: The self-assembly of 4,4′-bipyrimidine metal complexes into polymeric structures with distinct architectures demonstrates its potential in materials science. [, , , ]
- Computational Chemistry: Theoretical calculations provide valuable insights into reaction mechanisms, electronic properties, and structure-activity relationships, guiding the design and optimization of 4,4′-bipyrimidine-based materials and catalysts. [, , , , ]
Disclaimer and Information on In-Vitro Research Products
Please be aware that all articles and product information presented on BenchChem are intended solely for informational purposes. The products available for purchase on BenchChem are specifically designed for in-vitro studies, which are conducted outside of living organisms. In-vitro studies, derived from the Latin term "in glass," involve experiments performed in controlled laboratory settings using cells or tissues. It is important to note that these products are not categorized as medicines or drugs, and they have not received approval from the FDA for the prevention, treatment, or cure of any medical condition, ailment, or disease. We must emphasize that any form of bodily introduction of these products into humans or animals is strictly prohibited by law. It is essential to adhere to these guidelines to ensure compliance with legal and ethical standards in research and experimentation.