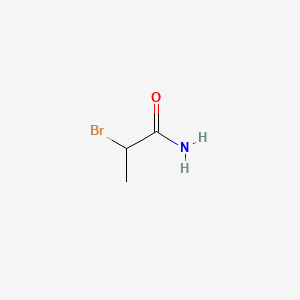
2-Bromopropanamide
Overview
Description
Preparation Methods
Synthetic Routes and Reaction Conditions: 2-Bromopropanamide can be synthesized through the bromination of propionamide. The reaction typically involves the use of bromine (Br2) in the presence of a catalyst such as phosphorus tribromide (PBr3) or hydrobromic acid (HBr). The reaction is carried out under controlled conditions to ensure the selective bromination at the α-position of the propionamide .
Industrial Production Methods: In industrial settings, the production of this compound may involve large-scale bromination processes with stringent control over reaction parameters to maximize yield and purity. The use of continuous flow reactors and advanced purification techniques such as recrystallization and distillation are common in industrial production .
Chemical Reactions Analysis
Types of Reactions: 2-Bromopropanamide undergoes various chemical reactions, including:
Substitution Reactions: It can participate in nucleophilic substitution reactions where the bromine atom is replaced by other nucleophiles such as amines, thiols, or alkoxides.
Common Reagents and Conditions:
Nucleophilic Substitution: Common reagents include sodium azide (NaN3), potassium thiocyanate (KSCN), and sodium methoxide (NaOCH3).
Hydrolysis: Acidic or basic conditions can be employed, with hydrochloric acid (HCl) or sodium hydroxide (NaOH) as catalysts.
Reduction: Reducing agents such as lithium aluminum hydride (LiAlH4) or sodium borohydride (NaBH4) are commonly used.
Major Products:
Nucleophilic Substitution: Products include substituted amides, thiols, and ethers.
Hydrolysis: Propionic acid and ammonium bromide.
Reduction: Propionamide and other reduced derivatives.
Scientific Research Applications
Pharmaceutical Industry
2-Bromopropanamide serves as an intermediate in the synthesis of several pharmaceutical compounds. Its applications include:
- Synthesis of Analgesics and Antipyretics: It is involved in creating drugs that alleviate pain and reduce fever.
- Opioid Research: Recent studies have highlighted its role in developing opioid analogs that exhibit potent analgesic properties. For example, compounds derived from this compound have shown significant binding affinity to the μ-opioid receptor, indicating potential for pain management therapies .
Chemical Research
In chemical research, this compound is valued for its utility in organic synthesis:
- Formation of Amides: It is used to synthesize various amides, which are crucial for developing peptides and other organic compounds.
- Reactivity with Nucleophiles: The compound's reactivity allows it to participate in diverse chemical reactions, facilitating the synthesis of complex molecules .
Agricultural Chemicals
The compound is also utilized in the agricultural sector:
- Synthesis of Pesticides and Herbicides: this compound contributes to the development of effective agricultural chemicals that enhance crop protection and yield .
Material Science
In material science, this compound finds applications in:
- Specialty Polymers: It is used to create polymers with specific chemical resistance and thermal stability, which are essential for various industrial applications.
Pharmaceutical Development
A notable study investigated the synthesis of novel propanamide derivatives using this compound as a precursor. The synthesized compounds exhibited promising anticancer activity, with some derivatives showing low IC₅₀ values comparable to established chemotherapeutics like doxorubicin . This reinforces the compound's potential as a building block for developing new therapeutic agents.
Agricultural Application
Research into the formulation of herbicides utilizing this compound has demonstrated its effectiveness in controlling weed populations while minimizing environmental impact. The compound's ability to enhance the efficacy of active ingredients has been highlighted in several studies focusing on sustainable agricultural practices .
Economic Impact
The economic implications of this compound are significant due to its role as an intermediate in various industrial processes. Its contributions to pharmaceutical development and agricultural innovation underscore its importance in driving growth within these sectors.
Mechanism of Action
The primary mechanism of action of 2-Bromopropanamide involves its role as a substrate in enzyme-catalyzed dehalogenation reactions. Enzymes such as dl-2-haloacid dehalogenase from Pseudomonas putida catalyze the hydrolytic dehalogenation of this compound, resulting in the formation of propionamide and bromide ions . This reaction is significant in bioremediation and the detoxification of halogenated compounds .
Comparison with Similar Compounds
2-Bromoacetamide: Similar in structure but with a different alkyl chain length.
2-Bromopropionitrile: Contains a nitrile group instead of an amide group.
2-Bromo-2-methylpropanamide: A branched analog with a methyl group at the α-position.
Uniqueness: 2-Bromopropanamide is unique due to its specific reactivity and the ability to undergo selective substitution reactions. Its role as a substrate in enzyme-catalyzed dehalogenation also sets it apart from other similar compounds .
Biological Activity
2-Bromopropanamide is a halogenated amide that has garnered interest in various fields of chemical research, particularly due to its potential biological activity. This compound is primarily studied for its applications in medicinal chemistry and its role as a synthetic intermediate in the preparation of more complex molecules. This article reviews the biological activity of this compound, focusing on its pharmacological properties, synthesis, and relevant case studies.
This compound is characterized by the presence of a bromine atom attached to a propanamide backbone. Its chemical structure can be represented as follows:
This structure facilitates various chemical reactions, making it a valuable compound in organic synthesis.
Antimicrobial Properties
Research indicates that this compound exhibits antimicrobial activity against various pathogens. A study highlighted its effectiveness against Trypanosoma cruzi, the causative agent of Chagas disease. The compound demonstrated a significant increase in potency compared to its acetamide analogs, suggesting that the bromine substitution enhances biological activity (Table 1) .
Compound | IC50 (µM) | Selectivity Index |
---|---|---|
This compound | 12.5 | 4.0 |
Acetamide Analog | 18.0 | 2.5 |
Cytotoxicity and Selectivity
In terms of cytotoxicity, this compound has been evaluated for its effects on mammalian cell lines. It was found to have lower toxicity levels compared to other halogenated amides, which enhances its therapeutic potential. The selectivity index (SI) indicates that while the compound is effective against parasites, it poses less risk to human cells .
Study on Enantioselective Reactions
A notable study explored the enantioselective reactions involving this compound with various amines. The results showed that this compound can be effectively utilized to synthesize chiral amides with high enantiomeric excess (ee). The dynamic kinetic resolution process achieved yields up to 95% ee, indicating significant potential for drug development .
Anticancer Activity
Another investigation focused on the synthesis of new propanamide derivatives bearing various substituents, including this compound. These derivatives were tested for anticancer activity against several cancer cell lines, revealing promising results. For instance, compounds derived from this compound exhibited IC50 values ranging from 10 µM to 25 µM, indicating strong anticancer potential .
The biological activity of this compound is believed to stem from its ability to interact with specific cellular targets. The bromine atom may enhance lipophilicity and facilitate membrane penetration, allowing for better interaction with intracellular targets such as enzymes and receptors involved in cell signaling pathways.
Q & A
Basic Research Questions
Q. What are the established synthetic routes for 2-bromopropanamide, and how can purity be optimized?
- Methodology: this compound is typically synthesized via nucleophilic substitution of propanamide with brominating agents (e.g., PBr₃ or HBr). Purity optimization involves fractional crystallization or column chromatography, validated by NMR (¹H/¹³C) and HPLC (>98% purity) .
- Key Considerations: Monitor reaction temperature (0–5°C to minimize side products) and stoichiometry (1:1.2 molar ratio of propanamide to bromine source).
Q. How can the structure of this compound be confirmed experimentally?
- Techniques: Use X-ray crystallography for absolute configuration determination, supplemented by FT-IR (C=O stretch at ~1680 cm⁻¹) and mass spectrometry (m/z 152/154 for [M+H]⁺ with Br isotope pattern) .
Q. What are the common reaction pathways involving this compound in organic synthesis?
- Pathways: Acts as an alkylating agent in nucleophilic substitutions (e.g., SN2 reactions with amines or thiols) and participates in cross-coupling reactions. Kinetic studies show rate acceleration in DNA-catalyzed systems (e.g., 100-bp DNA enhances reaction rates by 5–10× via 1D sliding mechanisms) .
Advanced Research Questions
Q. How do stereochemical and solvent effects influence the reactivity of this compound in enantioselective synthesis?
- Experimental Design: Compare (S)- and (R)-enantiomers using chiral HPLC. Polar aprotic solvents (e.g., DMF) stabilize transition states, while protic solvents (e.g., ethanol) reduce reaction rates. Stereoselectivity (>90% ee) is achievable with chiral ligands like BINAP .
- Data Analysis: Employ Eyring plots to quantify activation parameters (ΔH‡, ΔS‡) under varying solvent conditions .
Q. What strategies resolve contradictions in reported reaction yields for this compound-mediated alkylation?
- Approach: Replicate studies under standardized conditions (e.g., inert atmosphere, controlled humidity). Use kinetic isotope effects (KIEs) or computational modeling (DFT) to identify competing mechanisms (e.g., radical vs. ionic pathways) .
- Case Study: Discrepancies in DNA-catalyzed reactions may arise from DNA length (e.g., 100 bp vs. shorter fragments) or buffer composition (e.g., Mg²⁺ concentration affecting DNA rigidity) .
Q. How can this compound be integrated into multi-step syntheses of bioactive molecules?
- Workflow:
Step 1: Alkylate thiol-containing peptides (e.g., cysteine residues) for site-specific modifications.
Step 2: Couple with arylboronic acids via Suzuki-Miyaura reactions (Pd catalysis).
Step 3: Validate bioactivity using enzyme inhibition assays (e.g., IC₅₀ determination for kinase targets) .
- Analytical Validation: LC-MS/MS for tracking intermediates; circular dichroism (CD) for conformational analysis in DNA-interacting systems .
Q. Data Interpretation and Reproducibility
Q. What analytical methods distinguish between byproducts and desired products in this compound reactions?
- Techniques:
- GC-MS: Identify volatile byproducts (e.g., propionamide or dibrominated species).
- ²⁹Si NMR: Trace silane coupling agents in cross-coupling reactions.
- Crystallography: Resolve structural ambiguities in crystalline intermediates .
Q. How can researchers ensure reproducibility in DNA-accelerated reactions involving this compound?
- Best Practices:
- Standardize DNA preparation (e.g., sonication to ensure uniform length).
- Control ionic strength (e.g., 50 mM NaCl) and pH (7.4) to stabilize DNA-substrate interactions.
- Report raw kinetic data (e.g., time vs. product concentration plots) with error bars from triplicate trials .
Q. Contradiction Analysis and Hypothesis Testing
Q. Why do some studies report divergent catalytic efficiencies for this compound in DNA-based systems?
- Hypothesis Testing:
- Variable 1: DNA sequence/structure (e.g., AT-rich vs. GC-rich regions affecting sliding efficiency).
- Variable 2: Substrate stereochemistry (e.g., (R)-enantiomer may exhibit faster kinetics due to favorable DNA docking).
Q. How can conflicting results on this compound’s stability under acidic conditions be reconciled?
- Experimental Replication:
- Condition A: pH 2 (simulating gastric fluid): Monitor degradation via HPLC (t½ = 2 hours).
- Condition B: pH 5 (lysosomal environment): Stability increases (t½ = 8 hours).
- Mechanistic Insight: Acid-catalyzed hydrolysis follows first-order kinetics; stabilize via co-solvents (e.g., 10% DMSO) .
Q. Research Design and Validation
Q. What metrics define a robust experimental design for studying this compound’s reactivity?
- Criteria:
- Power Analysis: Sample size (n ≥ 3) to detect 20% effect size with α = 0.05.
- Controls: Include background reactions (no catalyst) and internal standards (e.g., deuterated analogs).
- Blinding: Randomize trial order to minimize bias .
Q. How should researchers address gaps in mechanistic understanding of this compound reactions?
Properties
IUPAC Name |
2-bromopropanamide | |
---|---|---|
Source | PubChem | |
URL | https://pubchem.ncbi.nlm.nih.gov | |
Description | Data deposited in or computed by PubChem | |
InChI |
InChI=1S/C3H6BrNO/c1-2(4)3(5)6/h2H,1H3,(H2,5,6) | |
Source | PubChem | |
URL | https://pubchem.ncbi.nlm.nih.gov | |
Description | Data deposited in or computed by PubChem | |
InChI Key |
AUHYZQCEIVEMFH-UHFFFAOYSA-N | |
Source | PubChem | |
URL | https://pubchem.ncbi.nlm.nih.gov | |
Description | Data deposited in or computed by PubChem | |
Canonical SMILES |
CC(C(=O)N)Br | |
Source | PubChem | |
URL | https://pubchem.ncbi.nlm.nih.gov | |
Description | Data deposited in or computed by PubChem | |
Molecular Formula |
C3H6BrNO | |
Source | PubChem | |
URL | https://pubchem.ncbi.nlm.nih.gov | |
Description | Data deposited in or computed by PubChem | |
DSSTOX Substance ID |
DTXSID101031970 | |
Record name | 2-Bromopropanamide | |
Source | EPA DSSTox | |
URL | https://comptox.epa.gov/dashboard/DTXSID101031970 | |
Description | DSSTox provides a high quality public chemistry resource for supporting improved predictive toxicology. | |
Molecular Weight |
151.99 g/mol | |
Source | PubChem | |
URL | https://pubchem.ncbi.nlm.nih.gov | |
Description | Data deposited in or computed by PubChem | |
CAS No. |
5875-25-2 | |
Record name | 2-Bromopropionamide | |
Source | CAS Common Chemistry | |
URL | https://commonchemistry.cas.org/detail?cas_rn=5875-25-2 | |
Description | CAS Common Chemistry is an open community resource for accessing chemical information. Nearly 500,000 chemical substances from CAS REGISTRY cover areas of community interest, including common and frequently regulated chemicals, and those relevant to high school and undergraduate chemistry classes. This chemical information, curated by our expert scientists, is provided in alignment with our mission as a division of the American Chemical Society. | |
Explanation | The data from CAS Common Chemistry is provided under a CC-BY-NC 4.0 license, unless otherwise stated. | |
Record name | 2-Bromopropanamide | |
Source | ChemIDplus | |
URL | https://pubchem.ncbi.nlm.nih.gov/substance/?source=chemidplus&sourceid=0005875252 | |
Description | ChemIDplus is a free, web search system that provides access to the structure and nomenclature authority files used for the identification of chemical substances cited in National Library of Medicine (NLM) databases, including the TOXNET system. | |
Record name | 5875-25-2 | |
Source | DTP/NCI | |
URL | https://dtp.cancer.gov/dtpstandard/servlet/dwindex?searchtype=NSC&outputformat=html&searchlist=402153 | |
Description | The NCI Development Therapeutics Program (DTP) provides services and resources to the academic and private-sector research communities worldwide to facilitate the discovery and development of new cancer therapeutic agents. | |
Explanation | Unless otherwise indicated, all text within NCI products is free of copyright and may be reused without our permission. Credit the National Cancer Institute as the source. | |
Record name | 2-Bromopropanamide | |
Source | EPA DSSTox | |
URL | https://comptox.epa.gov/dashboard/DTXSID101031970 | |
Description | DSSTox provides a high quality public chemistry resource for supporting improved predictive toxicology. | |
Record name | 2-bromopropanamide | |
Source | European Chemicals Agency (ECHA) | |
URL | https://echa.europa.eu/information-on-chemicals | |
Description | The European Chemicals Agency (ECHA) is an agency of the European Union which is the driving force among regulatory authorities in implementing the EU's groundbreaking chemicals legislation for the benefit of human health and the environment as well as for innovation and competitiveness. | |
Explanation | Use of the information, documents and data from the ECHA website is subject to the terms and conditions of this Legal Notice, and subject to other binding limitations provided for under applicable law, the information, documents and data made available on the ECHA website may be reproduced, distributed and/or used, totally or in part, for non-commercial purposes provided that ECHA is acknowledged as the source: "Source: European Chemicals Agency, http://echa.europa.eu/". Such acknowledgement must be included in each copy of the material. ECHA permits and encourages organisations and individuals to create links to the ECHA website under the following cumulative conditions: Links can only be made to webpages that provide a link to the Legal Notice page. | |
Record name | 2-BROMOPROPANAMIDE | |
Source | FDA Global Substance Registration System (GSRS) | |
URL | https://gsrs.ncats.nih.gov/ginas/app/beta/substances/92W215G050 | |
Description | The FDA Global Substance Registration System (GSRS) enables the efficient and accurate exchange of information on what substances are in regulated products. Instead of relying on names, which vary across regulatory domains, countries, and regions, the GSRS knowledge base makes it possible for substances to be defined by standardized, scientific descriptions. | |
Explanation | Unless otherwise noted, the contents of the FDA website (www.fda.gov), both text and graphics, are not copyrighted. They are in the public domain and may be republished, reprinted and otherwise used freely by anyone without the need to obtain permission from FDA. Credit to the U.S. Food and Drug Administration as the source is appreciated but not required. | |
Retrosynthesis Analysis
AI-Powered Synthesis Planning: Our tool employs the Template_relevance Pistachio, Template_relevance Bkms_metabolic, Template_relevance Pistachio_ringbreaker, Template_relevance Reaxys, Template_relevance Reaxys_biocatalysis model, leveraging a vast database of chemical reactions to predict feasible synthetic routes.
One-Step Synthesis Focus: Specifically designed for one-step synthesis, it provides concise and direct routes for your target compounds, streamlining the synthesis process.
Accurate Predictions: Utilizing the extensive PISTACHIO, BKMS_METABOLIC, PISTACHIO_RINGBREAKER, REAXYS, REAXYS_BIOCATALYSIS database, our tool offers high-accuracy predictions, reflecting the latest in chemical research and data.
Strategy Settings
Precursor scoring | Relevance Heuristic |
---|---|
Min. plausibility | 0.01 |
Model | Template_relevance |
Template Set | Pistachio/Bkms_metabolic/Pistachio_ringbreaker/Reaxys/Reaxys_biocatalysis |
Top-N result to add to graph | 6 |
Feasible Synthetic Routes
Disclaimer and Information on In-Vitro Research Products
Please be aware that all articles and product information presented on BenchChem are intended solely for informational purposes. The products available for purchase on BenchChem are specifically designed for in-vitro studies, which are conducted outside of living organisms. In-vitro studies, derived from the Latin term "in glass," involve experiments performed in controlled laboratory settings using cells or tissues. It is important to note that these products are not categorized as medicines or drugs, and they have not received approval from the FDA for the prevention, treatment, or cure of any medical condition, ailment, or disease. We must emphasize that any form of bodily introduction of these products into humans or animals is strictly prohibited by law. It is essential to adhere to these guidelines to ensure compliance with legal and ethical standards in research and experimentation.